IJCRR - 2(10), October, 2010
Pages: 16-26
Print Article
Download XML Download PDF
CONSTRUCTION OF CHIMERIC CRY2A GENE OF BACILLUS THURINGIENSIS BY DOMAIN SWAPPING AND ITS ANALYSIS
Author: Indra Arulselvi P, Udayasuriyan V
Category: General Sciences
Abstract:A chimeric cry2A gene of Bacillus thuringiensis (Bt) was constructed by domain swapping. DNA fragment corresponding to domain I & II of Cry2Aa and domain III of Cry2Ac was amplified by PCR and cloned individually in a separate pBluescript vector. The cloned DNA fragments of cry2A genes were aligned to construct a chimeric cry2Ax2 gene. The chimeric cry2Ax2 gene was cloned in an E.coli-Bt shuttle vector in
between cry3Aa promoter and cry2Aa terminator, for gene expression studies. Though the presence of chimeric cry2Ax2 gene has been verified by PCR, its expression in the transformants of acrystalliferous Bt strain, 4Q7, was not obvious in SDS-PAGE analysis. The non-expression of the chimeric cry2Ax2 gene may be attributed to the biasness of the new codons introduced during the process of its construction. Expression of the newly constructed chimeric cry2Ax2 gene may be achieved in the recombinant Bt strain after site directed mutagenesis or by constructing the gene by a suitable promoter in E. coli. After getting the chimeric gene expressed, it can be used for further bioassays to assess its toxicity against lepidopteron pest.
Keywords: Bacillus thuringiensis, chimeric cry2A gene, domain swapping, E. coli-Bt shuttle vector.
Full Text:
INTRODUCTION
Bacillus thuringiensis (Bt), is a wellknown gram-positive, spore-forming soil bacterium that forms parasporal insecticidal crystal proteins during the stationary phase of its growth cycle. These proteins are termed deltaendotoxins because of their intracellular location and have been used for many years as successful biological insecticides1 . Cloning of the first crystal protein gene (cry) of Bt was reported by Schnepf & Whiteley2 , since then more than 270 cry genes have been cloned, characterized, and their classification based on amino acid sequence similarity of their proteins3 . Cloning of cry genes provides an opportunity to express the cloned gene in acrystalliferous Bt or E. coli to find out the insecticidal activities of their proteins. In the natural isolates of Bt the cry2Aa and cry2Ac genes are expressed as third orf in operon model whereas cry2Ab gene is cryptic in nature. Dankocsik et al. 4 achieved expression of cry2Ab gene by the promoter of cry3Aa gene in an acrystalliferous strain of Bt. Transgenic crops that produce Cry1A toxin can control some key lepidopteran pests5 . Continuous exposure to a single kind of Bt toxin can lead to resistance development in insect pests. Routine replacement of cry genes or pyramiding of cry genes could be useful for effective control of insect pests by transgenic technology. The Cry2A proteins of B. thuringiensis are promising candidates for management of resistance development in insects due to its differences from the currently used Cry1A proteins, in structure6 and mode of action7 . Combination of Cry1Ac and Cry2Ab is used in the second version of Bt cotton (Bollgard® II) in USA and Australia. But the Indian populations of H. armigera were thirtyfive fold less susceptible to Cry2Aa than Cry1Ac8 . Studies are insufficient on susceptibility of Indian population of H. armigera to different Cry2A proteins. Variation of a single amino acid can significantly influence the level of toxicity in Cry proteins9,10 . New variants of the already known cry gene subgroups11 , mutated12,13 and chimeric13 cry gene sequences could encode crystal proteins with significant difference in the level of toxicity due to variation in their sequences. Hence, in the present study a new chimeric cry2A gene was constructed and analysed.
MATERIALS AND METHODS
Genomic DNA isolation and amplification of Cry2A domains
Plasmid DNA was isolated from recombinant E. coli strains, harbouring p2Aa and p2Ac plasmids. The p2Aa plasmid contains cry2Aa operon and p2Ac plasmid contains cry2Ac operon. The plasmid DNA isolated from p2Aa and p2Ac plasmids was used as a template for PCR amplification. DNA fragments of ~1465 bp and~441 bp was amplified using 2AFS & 2AR2C and 2CF2C & 2ARS primers (Table 1). The PCR was performed for 30 cycles as follows: 94 °C for 1 min, 60 °C for 45 sec and 72 °C for 1.0 min., the final extension was performed for 7 min at 72 °C. The amplicons were column purified and examined on 1.2 % agarose gel.
Cloning of amplicons individually in pBKS vector Two sets of pBKS vector
were linearized by double digestion with KpnI and HindIII for one set and HindIII and XbaI for another set. The amplicon of ~1465 bp (domain I & II of Cry2Aa) was double digested with KpnI and HindIII. The amplicon of ~441 bp (domain III of Cry2Ac) was also double digested with HindIII and XbaI. The digested vector and the amplicons were resolved in agarose gel, excised from the gel and purified by gel extraction kit. Two sets of ligation mixture were prepared. The pBluescript vector digested with KpnI and HindIII
restriction enzymes and the insert DNA fragment of ~1465 bp digested by the same enzymes were used in one set of ligation. In another set of ligation mixture, the pBluescript vector digested with HindIII and XbaI restriction enzymes and the insert DNA fragment of ~441 bp digested by the same enzymes were used. The vector and the insert DNA was used in 1: 3 ratio for ligation reaction. Ligation mixture was prepared and transformed to E.coli cells. The transformed colonies were selected on LB plate with XIA. The white colonies were screened by colony PCR with respective primers for checking the presence of insert. Restriction digestion was carried out as per the manufacturer?s instruction. The recombinant pBKS plasmids (containing DNA fragment corresponding to domain I&II of Cry2Aa and DNA fragment corresponding to domain III of Cry2Ac) was double digested by KpnI & HindIII and HindIII & XbaI, respectively to release the inserts. The digested product was kept at -20ºC to stop the reaction and analyzed by agarose gel electrophoresis.
Aligning of cloned DNA fragments to construct the chimeric gene, cry2Ax2
The recombinant pBKS containing DNA fragment corresponding to domain I & II of Cry2Aa was double digested by HindIII & XbaI for cloning domain III of Cry2Ac between HindIII & XbaI sites. The linearized recombinant pBKS harboring domain I & II of Cry2Aa and the insert (~441 bp of domain III of Cry2Ac) were excised from 0.8 % agarose gel. The DNA fragments were extracted using gelextraction kit. Recombinant pBluescript vector and insert (DNA fragment of ~441 bp corresponding to domain III of Cry2Ac) were ligated used for transformation of E. coli. The transformants of E. coli colonies were screened by colony PCR and further confirmed by double digestion with KpnI & XbaI restriction enzymes.
Cloning of the chimeric cry2Ax2 gene in expression vector pHT3P2T
The recombinant pBKS plasmid carrying chimeric gene cry2Ax2 was double digested by KpnI and XbaI restriction enzymes to release the cloned DNA fragment. Simultaneously the expression vector pHT3P2T was also double digested using the same enzymes to linearize it. The expression vector pHT3P2T and insert (cry2Ax2) was ligated and transformed into E. coli. The presence of chimeric gene cry2Ax2 was confirmed by restriction digestion of the recombinant pHT3P2T plasmid with the KpnI and XbaI enzymes.
Transformation, screening and analysis of Bt strain 4Q7
Plasmid was isolated from recombinant E.coli colonies and transformed to acrystalliferous Bt strain, 4Q7 through electroporation15. Transformed Bt colonies were selected on LB agar plate containing erythromycin (50 µg/ml). The Bt transformants were screened for the presence of the chimeric gene cry2Ax2 by PCR using 2AFS and 2AR2C primers (specific for DNA fragment corresponding to domain I and II of the chimeric gene cry2Ax2) and 2CF2C and 2ARS (specific for DNA fragment corresponding to domain III of the chimeric gene cry2Ax2). Spore crystal mixture was prepared from recombinant Bt strain, 4Q7 and analysed by SDS-PAGE.
RESULTS
Amplification and cloning of DNA fragment corresponding to domain I & II of Cry2Aa
domain III of Cry2Ac The DNA fragment of about 1465 bp encoding domain I & II of Cry2Aa was amplified from p2Aa with 2AFS and 2AR2C primers by PCR. The ~1465 bp amplicon was subjected for double digestion with KpnI and HindIII. The double digested ~1465 bp amplicon was cloned into the vector pBKS. Recombinant E. coli clones were further screened for the presence of cloned DNA fragment by colony PCR using gene specific primers of the DNA fragment corresponding to domain I & II of Cry2Aa and by restriction digestion. The resultant plasmid is designated as pBKS2AA. The DNA fragment of about 441 bp encoding domain III of Cry2Ac was amplified from p2Ac with 2CF2C and 2ARS primers by PCR. The amplicon (~441 bp) was subjected for double digestion with HindIII and XbaI and cloned into the pBKS vector. Recombinant E. coli clones were further screened for the presence of cloned DNA fragment by colony PCR using gene specific primers of the DNA fragment corresponding to domain III of Cry2Ac and by restriction digestion. The resultant plasmid is designated as pBKS2AC.
Aligning of DNA fragments corresponding to domain I & II of Cry2Aa and domain III of Cry2Ac The recombinant pBKS2AA and pBKS2AC plasmids were double digested with KpnI & HindIII and HindIII & XbaI, respectively. The released insert of ~441 bp from pBKS2AC plasmid was cloned into the linearized recombinant plasmid, pBKS2AA. The recombinant colonies were screened by colony PCR for the presence of gene specific primers of the DNA fragment corresponding to domain I & II of Cry2Aa and domain III of Cry2Ac and by restriction digestion (Fig. 1A). The constructed chimeric gene and its plasmid is named as cry2Ax2 and pBKS2Ax2, respectively. Alignment of nucleotide sequence data obtained from chimeric cry2Ax2 gene with that of cry2Aa1 and cry2Ac1 genes indicated fusion of domain I & II of Cry2Aa and domain III of Cry2Ac in the expected manner.
Cloning of chimeric cry2Ax2 gene in expression vector, pHT3P2T
The recombinant pBKS2Ax2 and expression vector pHT3P2T were double digested with KpnI and XbaI restriction enzymes. The released insert of ~1.9 kb from pBKS2Ax2 plasmid was cloned into the linearized recombinant plasmid, pHT3P2T. The recombinant colonies were confirmed by restriction digestion (Fig. 1B). The recombinant pHT3P2T plasmid harboring cry2Ax2 is named as p2Ax2.
Transformation of Bt strain 4Q7 with p2Ax2
The p2Ax2 plasmids (containing cry2Ax2 gene under the transcriptional control of cry3Aa promoter and cry2Aa terminator) was transformed into the acrystalliferous Bt strain 4Q7 and selected on LB agar plate containing erythromycin (50 µg/ml). Transformants of Bt strain, 4Q7 were screened by PCR using gene specific primers. The amplification of DNA fragments corresponding to domain I & II of Cry2Aa (~1.5 kb) and domain-III of Cry2Ac (~441 bp) was observed in the transformants of Bt strain 4Q7 and there was no amplification in the case of negative control, 4Q7.
Comparison of protein profile of recombinant 4Q7 strains harboring cry2Aa and cry2Ax2 gene expression plasmids
The spore-crystal mixtures obtained from the recombinant Bt strain, 4Q7 harbouring cry2Aa and cry2Ax2 genes under the transcriptional control of cry3Aa promoter and cry2Aa terminator were subjected to SDS-PAGE analysis. The transformants of Bt strain 4Q7 harboring p2Ax2 plasmid did not show any prominent band equivalent to the size of Cry2Aa protein (~65 kDa) where as other positive control, recombinant Bt strain harbouring pHT3P2AT plasmid (Cry2Aa protein), showed the prominent band of ~65 kDa. The protein profile of the recombinant 4Q7 (pHT2Ax2) harboring the chimeric cry2Ax2 gene was similar to that of negative control 4Q7 as shown in fig. 2.
DISCUSSION
A chimeric gene was constructed by replacing the whole DNA fragment of 441 bp encoding domain III of Cry2Ac with that of Cry2Aa. De Maagd et. al.,18 had shown that several Cry1 toxins (e.g. Cry1Ab, Cry1Ac, Cry1Ba, Cry1Ea) with low or no activity against beet armyworm (Spodoptera exigua) become active when their domain III is replaced by that of Cry1Ca. Alternatively, Malvar and Gilmer 19 showed that hybrids of Cry1Ac and Cry1Fa have a wider target spectrum than either of the parental toxins from which they were derived. Rang et al., 20 , demonstrated that domain-III appears to modulate the activity of the chimeric toxins. They also explained that combination of domain -III from Cry1Ab with domain-I and II of Cry1C showed strong activity against insect cell lines than wild type Cry1C. Domain-III of Cry1Ac is involved in the specificity of binding to the putative Cry1Ac receptor from Manduca sexta, aminopeptidase, as well as in the binding to intact membranes 18 . The cloning of DNA fragment of 1465 bp encoding domain I & II of Cry2Aa in pBKS was achieved by domain I & II specific primers by introducing KpnI and HindIII restriction sites at 5? and 3? end respectively. The cloning of DNA fragment of 441 bp encoding domain III of Cry2Ac in pBKS was achieved by its domain specific primers by introducing HindIII and XbaI restriction sites 5? and 3? respectively. HindIII restriction site was created by changing the codons at 485 and 486 positions (CATTTG ? AAGCTT) there by altering the amino acids at 485th position (His ?Lys). The chimeric gene of ~1.9 kb was constructed by aligning domain III of cry2Ac at 5? end between HindIII and XbaI sites of recombinant pBKS containing domain I & II fragment of cry2Aa. The construction of chimeric gene was verified by sequencing and named as cry2Ax2. The cry3Aa gene, isolated from the coleopteran active B. thuringiensis var. tenebrionis19, is a typical example of a non-sporulation-dependent cry gene. It has been shown that the cry3Aa promoter is weak, but significantly expressed during vegetative phase of growth unlike the cry1A promoter, which is sporulation dependent. The expression of cry3Aa is not dependent on sporulation-specific sigma factors either in B. subtilis 21 or in B. thuringiensis 22. The cry3A promoter, although located unusually far upstream of the start codon (position - 558), resembles promoter recognized by the primary sigma factor (σA ) of vegetative cells. Moreover, cry3A expression is increased and prolonged in mutant strains, which are unable to initiate sporulation 23 . In the present study the constructed chimeric gene cry2Ax2 was cloned into expression shuttle vector pHT3PT between cry3Aa promotor and cry2A terminator. In the previous studies the vector has been tested for expression of cry2Aa gene24. The recombinant shuttle vector containing the chimeric gene cry2Ax2 was transferred to Bt strain 4Q7 by electroporation. SDS-PAGE analysis was done for spore crystal mixture harvested from the recombinant clones for Bt strain, 4Q7. Expression of the chimeric gene, cry2Ax2 was not seen as the prominent band of ~65 kDa. Protein profile of the recombinant 4Q7 was same as that in the case of negative control 4Q7. Positive control including recombinant 4Q7 containing cry2Aa gene in the same cassette showed expression as a prominent band of ~65 kDa. The non-expression of the chimeric gene, cry2Ax2, may be attributed to usage of the codons that was introduced to create HindIII restriction site (CATTTG ? AAGCTT) for cloning DNA fragments. The usage for these two codons was compared with the codon usage table for B. thuringiensis from CODON USAGE DATABASE (http://www.kazusa.or.jp/codon/). The usage for CAT codon, which is coding for histidine amino acid was 12.6 and 16.1 per 1000 codons for cry2Aa and cry2Ac genes, respectively. For TTG codon, which is coding for leucine amino acid, the usage was 14.2 and 9.6 per 1000 codons for cry2Aa and cry2Ac genes, respectively. The usage of codons present in HindIII site showed that AAG codon, coding for lysine amino acid, was 1.6 per 1000 codons for both cry2Aa and cry2Ac genes. Another codon, CTT present in the HindIII site, coding leucine amino acid, showed usage of 18.9 and 28.9 per 1000 codons for cry2Aa and cry2Ac genes, respectively. Previous studies on codon usage showed that the frequencies with which individual synonymous codons are used to code their cognate amino acids is quite variable from genome to genome and within genomes, from gene to gene. There is general agreement that codons are translated at different rates25. Hence codon usage has been identified as the single most important factor in prokaryotic gene expression26. In the present study, the introduced codons in the HindIII site of the chimeric cry2Ax2 gene is less preferred for Bt. Therefore, tRNA recognizing the anti-codons in mRNA during translation may not be present in sufficient amount for successful expression of the chimeric gene in the recombinant Bt strain.
CONCLUSION
The expression studies of the newly constructed chimeric cry2Ax2 gene may be done after changing the introduced codons back to the most preferred one (CATTTG ? AAGCTT) by site directed mutagenesis. In previous study, silent mutation of a less preferred codon for an amino acid to more preferred one have increased the expression to four fold27 . Expression of the newly constructed chimeric cry2Ax2 gene may be achieved in the recombinant Bt strain after site directed mutagenesis. The cry2A genes are known to be expressed in recombinant E. coli strains28,29. The codons introduced in the present study are not less preferred by E. coli. Therefore, expression of the newly constructed gene by a suitable promoter in E. coli may also be successful. After getting the chimeric gene expressed, it can be used for further bioassays to assess its toxicity against lepidopteron pest.
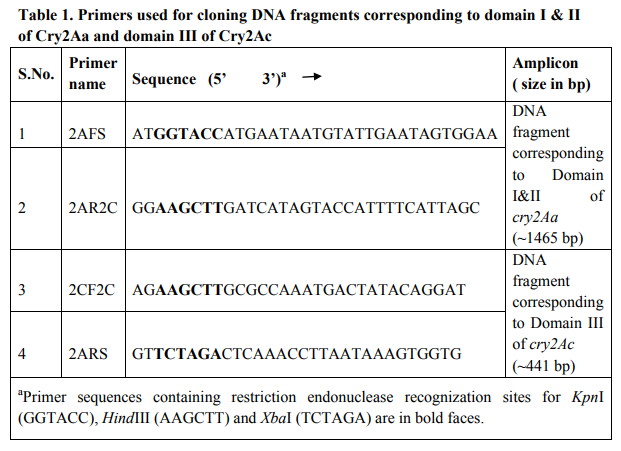
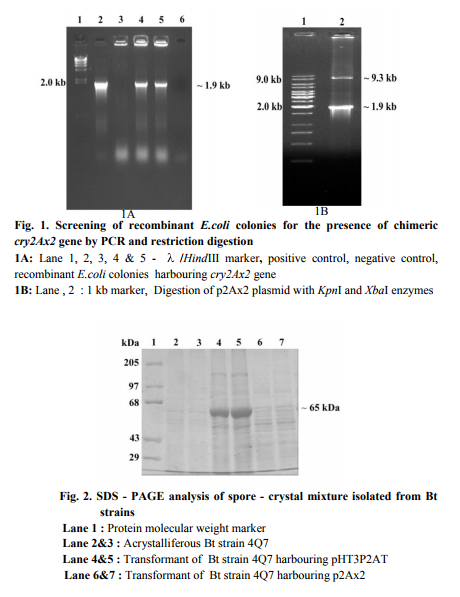
References:
1. Schnepf E, Crickmore N, Van Rie J, Lerecurs D, Baum J, Feitelson J, et al. B. thuringiensis and its pesticidal crystal proteins. Microbiol Mol Biol Rev 1998; 62: 775-806.
2. Schnepf HE and Whiteley HR. (ed.) Hoch JA and Setlow P Protein toxins of Bacilli. In: Molecular Biology of Microbial Differentiation.1981; Proc. Natl. Acad. Sci. USA, 78: 2989- 2897.
3. Crickmore ND, Zeigler R, Feitelson J, Schnepf E, Van Rie J, Lereclus J. et al. thuringiensis and its pesticidal crystal proteins. 1998; Microbiol. Mol. Biol. Rev., 62: 807-813.
4. Dankocsik C,Donovan WP and Jany CS. Redesigning Bacillus thuringiensis Cry1Aa Toxin into a Mosquito Toxin. 1990 ; Mol.Microbiol. 4:2087-2094.
5. Barton K.A., Whiteley HR and Yang NS. B. thuringiensis δ-endotoxin expressed in transgenic Nicotiana tabaccum provides resistance to lepidopteran insects. 1987; Plant Physiol., 85: 1103–1111.
6. Morse RJ, Yamamoto T and Stroud RM. Structure of Cry2Aa suggests an unexpected receptor binding epitope. 2001; Structure, 9 409-417.
7. English L, Robbins HL, Von Tersch MA, Kulesza CA, Ave D, Coyle D, et al. Mode of action of CryIIA: a B. thuringiensis delta-endotoxin. 1994 ;Insect Biochem. Molec. Biol., 24: 1025-10358.
8. Chakrabarti SK , Mandaokar A, Kumar AP and Sharma RP. Insect resistant transgenic plants. 1998; J. 23 International Journal of Current Research and Review www.ijcrr.com Vol. 02 issue 10 Oct 2010 Invertebr. Pathol., 72: 336-337.
9. Udayasuriyan V, Nakamura A, Mori.A, Masaki H and Uozumi T. Cloning of a new crylA (a), gene from B. thuringiensis strain FU- 2-7 and analysis of chimeric cry1A(a) proteins of toxicity. 1994; Biosci. Biotechnol. Biochem., 58: 830-835.
10. Rajamohan FO, Alzate JA, Cotrill A. Curtiss and Dean DH. Protein engineering of Bacillus thuringiensis δ Endotoxin: Mutations at domain II of Cry1Ab enhance receptor affinity and toxicity towards gypsy moth larvae. 1996; Proc. Natl. Acad. Sci. USA, 93: 14338-14343.
11. Sasaki J, Asano S, Hashimoto N, Lay BW, Hastowo S , Bando H ,et al. Characterization of a cry2A gen cloned from an isolate of B. thuringiensis serovar sotto 1997; Curr. Microbiol., 35: 1-8
. 12. Chandra A, Ghosh P, Mandoakar AD, Bera AK , Sharma RP, Das R et al. Amino acid substitution in α?helix7 Cry1Ac δ endotoxin of Bacillus thuringiensis leads to enhanced toxicity to Helicoverpa armigera Hubner. 1999; FEBS Letters, 458:174-179.
13. Karlova R, Weeman-Hendriks M, Naimov S, Ceron J, Dukiandjiev S and de Maagd RA . Bacillus thuringiensis δ- endotoxin Cry1Ac domain–III enhances activity against Heliothis virescens in some, but not all Cry 1-Cry1Ac hybrids. 2005;J Invertebr Pathol., 88: 169- 172.
14. Sambrook J, Fritsch EF and Maniatis T. 1989; Molecular Cloning: A Laboratory Manual, 2nd Edition, Cold Spring Harbour Laboratory, New York. ISBN 0- 87969-309-6.
15. Mahillon J , Chungjatopornchai W, Decock S, Dierickx F, Michiels M. Peferoen and Joos H. Transformation of Bacillus thuringiensis by electroporation. 1989 FEMS Microbiology Letters, 60: 205-210.
16. De Maagd R, Weemen-Hendriks AM , W. Stiekema and D. Bosch. Cross-resistance of pink bollworm (P. gossypiella) to B. thuringiensis toxins. (2000). Appl. Environ. Microbiol., 66: 1559-1563.
17. Malvar T and Gilmer A. Broadspectrum delta-endotoxin s 1998; Patent WO 98/22595 World Intellectual Property Organization.
18. De Maagd RA, Bosch D and Stickema W. Interaction between functional domains of Bacillus thuringiensis insecticidal crystal proteins 1999; B. thuringiensis toxin mediated insect resistance in plants. Science, 4: 9-13. 19. Sekar V, Thompson DV, Maroney JM, Bookland RG and Adang MJ. Molecular cloning and
characterization of the insecticidal crystal protein gene of B. thuringiensis var. tenebrionis. 1987; Proc. Natl. Acad. Sci. USA, 84: 7036-7040.
20. Rang C, Vachon V, de Maagd A, Villalon M, Schwartz JL, Bosch D,et al. 1999; Interaction between functional domains of Bacillus thuringiensis insecticidal crystal 24 International Journal of Current Research and Review www.ijcrr.com Vol. 02 issue 10 Oct 2010 proteins. Appl. Environ. Microbiol., 65: 2918-2925
. 21. Agaisse H and Lereclus D. Expression in B. subtilis of the B. thuringiensis cryIIIA toxin gene is not dependent on a sporulation specific sigma factor and is increased in a spo0A mutant. 1994; J. Bacteriol., 176: 4734– 4741.
22. Salamitou S, Agaisse H, Bravo A and Lereclus D. Genetic analysis of cryIIIA gene expression in B. thuringiensis.1996; Microbiology, 142: 2049– 2055.
23. Malavar T and Baum J. Tn5401 disruption of the spo0F gene, identified by direct chromosomal sequencing, results in cryIIIA overproduction in B. thuringiensis. 1994 ;J. Bacteriol., 176: 4750– 4753.
24. Jain D. Cloning of cry2Ab gene and construction of plasmids for expression of indigenous cry2A genes 2005; Unpublished M.Sc. thesis submitted to the Tamil Nadu Agricultural University, Coimbatore, India, and 121 p.
25. Khurland CG. Codon bias and gene expression 1991; FEBS Lett., 285; 165–169.
26. Lithwick G and Margalit H. Relative predicted protein levels of functionally associated proteins are conserved across organisms 2003; Genome Res., 13: 2665–2673.
27. Klasen M and Wabl M. Silent point mutation in DsRed resulting in enhanced relative fluorescence intensity. 2004; Biotechniques., 36: 236–237.
28. Shantanu Kumar. 2003; Cloning and expression of cry2Aa and cry2Ab genes from new isolates of Bacillus thuringiensis and insecticidal analysis of their proteins. Unpublished Ph. D thesis submitted to the Tamil Nadu Agricultural University, Coimbatore, India, and 121 p.
29. Promdonkoy B, Promdonkoy P and Panyim S. Co-expression of Bacillus thuringiensis Cry4Ba and Cyt2Aa2 in Escherichia coli revealed high synergism against Aedes aegypti and Culex quinquefasciatus larvae. 2005; FEMS Microbiology Letters, 252: 121–126.
|