IJCRR - 3(3), March, 2011
Pages: 22-33
Print Article
Download XML Download PDF
LECITHIN-MICROEMULSION BASED ORGANOGELS AS TOPICAL DRUG DELIVERY SYSTEM (TDDS)
Author: Md. Akhlaquer Rahman, Arshad Hussain, Md. Sarfaraj Hussain, L. Ratnakar Singh
Category: Healthcare
Abstract:Most of the chemical entities that are being discovered are lipophilic in nature and have poor
aqueous solubility, there by posing problems in their formulation into delivery systems.
Because of their low aqueous solubility and high permeability, dissolution and/or release rate
from the delivery system forms the rate-limiting step in their absorption and systemic
availability. This frequently results in potentially important products not reaching the market or
not achieving their full potential. Transdermal drug delivery provides the promising delivery
system for those drugs. It provides better patient compliance by avoiding invasiveness,
prolonging plasma drug level, bypass first pass effect, reduced side effects and easy termination
of therapy. Interest in organogels has increased in a wide variety of fields including chemistry,
biotechnology and pharmaceutics. It is more reasonable to look for a carrier that interacts with
the skin such that it allows various molecules to pass into the skin. In this paper, which follows
a review on investigation of lecithin organogels as carriers for the transdermal transport of
drugs having problems mentioned above. The general properties of lecithin organogels, method
of preparation and its characterization have been discussed. The potential use of lecithin
organogels for the transdermal transport of many therapeutic agents have also been discussed in
the present review.
Keywords: Transdermal Drug delivery; Lecithin organogels; Release rate; Microemulsion-based gels; Rheology
Full Text:
INTRODUCTION
The skin is an exceptionally effective barrier to most drugs for therapeutic treatment. Very few drugs in therapeutic amount are permeated through skin such as nitroglycerine, scopolamine, nicotine, clonidine, fentanyl, estradiol, testosterone, Lidocaine, and oxybutinin [1]. Therefore, the systems that make the skin more permeable and thereby enhance transdermal delivery are of great formulation interest. The strategies to deliver the medicament into the skin and for systemic circulation have been evolved. The extensive research has been reported on lipids as skin penetration enhancers [2- 5]. Lipids in the form of vesicles such as liposomes, niosomes [6-8], ethosomes [9] and transfersomes [10] have been evaluated. The lipid-based formulations have been in use since decades. The importance of lipids has especially increased after realizing the utility of natural phospholipids. Lecithin, the natural biofriendly molecules are ubiquitous phospholipids that accounts for more than 50% of the lipid matrix of biological membranes. Soybean lecithin is an apolar organic solvent, on addition of water, forms an entangled dynamic network of long and flexible worm like multi-molecular aggregates termed as „organogels? [11]. These are characterized by high viscosity and complete optical transparency. Lecithin organogels are emerging as carriers for drug molecules with diverse physicochemical properties including macromolecules [12]. Transdermal transport rates of scopolamine and broxaterol from lecithin organogels were faster than commercial patches [12]. With the advent of high throughput screening techniques, the discovery of biologically active molecules is taking place at a pace never seen before. Most of the chemical entities that are being discovered are lipophilic in nature and have poor aqueous solubility, there by posing problems in their formulation into delivery systems. Because of their low aqueous solubility and high permeability, dissolution and/or release rate from the delivery system forms the rate-limiting step in their absorption and systemic availability. More than 60% of potential drug products suffer from poor water solubility. This frequently results in potentially important products not reaching the market or not achieving their full potential. Pharmaceutical industry is quick in realizing the importance of solubility and dissolution rate in bioavailability and good deal of research has been done in this area. Currently a number of technologies are available to address the poor solubility, dissolution rate and bioavailability of insoluble drugs [13]
Conventional Technologies Conventionally used techniques [14] based on Noyes-Whitney equation [15] for enhancing solubility, dissolution rate and thereby bioavailability of insoluble drugs include buffered tablets, use of salts, solvates and hydrates, polymorphic forms, complexation, prodrugs, micronisation, solid dispersions and solvent deposited systems.
Newer Technologies Newer and novel drug delivery technologies developed in recent years for bioavailability enhancement of insoluble drugs are described below.
Lipid Based Delivery Systems Lipid emulsion technology [16], Selfemulsifying drug delivery system [17], Micro emulsion media as novel drug delivery system [18]
Microemulsion System
Microemulsions are four component mixtures composing of an oil phase, a water phase surfactant/s and a cosurfactant. The tendency towards formation of w/o or o/w microemulsions is dependent on the properties of the oil and the surfactant, the water-to-oil-ratio and the temperature. When a mixture of surfactant and co-surfactant is added to a biphasic oilwater system, a thermodynamically stable, optically transparent or translucent, low viscosity and isotropic mixture spontaneously forms. The transparency of these systems arises from their small droplets diameter (10-100 nm). Such small droplets produce only weak scattering of visible light when compared with that from the coarse droplets (0.5-100 µm) of traditional or standard macroemulsions such as emollient liquids, cream, lotions, etc., Structurally, microemulsions have normal micellar solutions, reverse micelles, cores or droplets of water or oil, and, for some systems, even bicontinuous structures could solubilize large amounts of both oil and water soluble drugs within microemulsions. There is rather confusing situation in the medical literatures, where the term “microemulsion” is indifferently used to indicate systems of presumably unlike structure (“true” microemulsions and miniemulsions). Some studies have compared the performance of different emulsified systems (macroemulsions, microemulsion, multiple emulsion and gelemulsions) prepared with similar oils and surfactants for applications such as controlled drug release or drug protection. The surfactants used to stabilize such systems may be (i) Non-ionic (ii) Zwitterionic (iii) Cationic (iv) Anionic surfactants. Combinations of these, particularly ionic and non-ionic, can be very effective at increasing the extent of the microemulsion region [18,19].
Rational Approach to Drug Delivery To & Via Skin There are three main ways to solve the problem of formulating a successful topical dosage formulation [20]. 1. We can manipulate the barrier function of the skin e.g., topical antibiotics and antibacterials help a damaged barrier to ward-off infection, sunscreen agents and the horny layer protect the viable tissue from ultraviolet radiation and emollient preparations restore palatability to a desiccated horny layer. 2. We can direct drug to the viable skin tissue without using oral, systemic or other route of therapy. 3. The third approach uses skin delivery for systemic treatment. For example, topical drug delivery systems provide systemic therapy for conditions such as motion sickness, angina and pain. Dermatologists aim at five main target region–skin surface, horny layers, viable epidermis and upper dermis, skin glands and systemic circulation.
Emulsion-Gels as Topical Formulations
Transdermal and topical formulations are becoming increasingly important and their use in therapy is becoming more widespread. But the skin acts as a barrier to topically administered drugs. Attempts have been made to circumvent the skin barrier by several means, emulsion-gels being one such promising technique [21].
Organogels The topical administration of drugs in order to achieve optimal cutaneous and percutaneous drug delivery has recently gained an importance because of various advantages such as ease of administration, non-invasive, better tolerated and compliance, local enhanced transdermal delivery, avoidance of local gastrointestinal toxicity, avoidance of first pass metabolism. In search of a vehicle to deliver the medicament into the skin layer (cutaneous delivery) or through the skin and into systemic circulation (percutaneous absorption) and to target the skin, varied kind of formulation systems and strategies have been evolved. Amongst the many, the lipid-based formulations have been in use since decades. Pharmaceutically, lipid emulsions may allow the sustained release of drugs by sink mechanism [19]. The importance of lipids has especially increased after realizing the utility of phospholipids. The natural bio-friendly molecules which in collaboration with water can form diverse type of polymolecular/super molecular structure with retardant release in sustained release formulation [22] The topical delivery has been attempted and made successful using a number of lipid based systems viz., vesicular systems [23], lipid microsphere, lipid nanoparticles [13], lipid emulsion [16], polymeric gels [24]. In a recent development, phospholipids in conjunction with some other additives have been shown to provide a very promising topical drug delivery vehicle i.e., lecithin organogel. Lecithin organogels (LOs) are thermodynamically stable, clear, viscoelastic, biocompatible and isotropic gels composed of phospholipids (lecithin), appropriate organic solvent and a polar solvent [25]. Lecithin organogel, the jelly like phase consists of three dimensional network of entangled reverse cylindrical (polymer like) micelle, which immobilize the continuous or macroscopic external organic phase, thus turning liquid into a gel [22]. The formation of three-dimensional network in the organogel is the result of transition at the micellar level in a low viscous network liquid consisting of lecithin cause micelles in non-polar organic liquid [26]. This spherical reverse micellar state of lipid aggregates, twins on to form elongated tubular micelles with the addition of water, and subsequently entangle to form a temporal three dimensional network in the solution bulk. The latter serves to immobilize the external organic phase, thus producing a gel form or the jelly like state of the initial non-viscous solution. However, the transparency and optical isotropy of the organogel remain as before. For this reason, these systems are often called as polymer like micelles and are also termed as living or equilibrium polymer, worm like or thread like micelles [22].
Advantages of Organogels as Topical Delivery Potential [27,28]
Being well balanced in hydrophilic and lipophilic character, they can efficiently partition with the skin and therefore enhance the skin penetration and transport of the molecules. Lecithin organogels also provide the desired hydration of skin in a lipidenriched environment so as to maintain the bioactive state of skin. Lecithin might influence the structure of the skin by disorganizing the lipid layer in the stratum.
Limitations of Organogels In the lecithin organogels, the lecithin should be pure otherwise no gelling will
occur.
Lecithin is most costly. Lecithin is not available on large scale. Should be stored in a proper condition. The organogel has greasy property. Less stable to temperature.
Organogelling Composition
The organogel matrix chiefly consists of surfactant (lecithin) as gelation molecules, a non-polar organic solvent as external or continuous phase and polar agent, usually water. Lecithin is a trivial name for 1,2- diacyl-Sn-3-phosphocholine. It belongs to a biological essential class of substance termed phosphoglycerides or phospholipids. The latter form the lipid matrix of biological membrane and also play a key role in the cellular metabolism. As a biocompatible surfactant, it is widely used in everyday life including human and animal food, medicine, cosmetics and manifold industrial applications [29]. Synthetic lecithin containing residues of saturated fatty acids failed to form organogel [27,30]. However, it has been established that unsaturation in phospholipid molecules is a desired property for the formation of lecithin organogels. Besides lecithin as gelation molecules, the role of organic solvent in providing the desired solvent action into the gelatin molecules is much emphasized. A large variety of organic solvent are able to form gel in the presence of lecithin. Among them are linear, branched and cyclic alkenes, ethers and esters, fatty acids and amines. Specific examples includes ethyl laurates, ethyl myristate, isopropyl myristate (IPM), isopropyl palmitate (IPP), cyclopentane, cycloclane, n-pentane, nhexane, n-hexadecane and tripropylamine [25].
Amongst the above, the fatty acid esters i.e., application of lecithin organogels. This has been attributed to their skin penetration enhancing property besides their biocompatible and biodegradable nature [29,31].
The third component of polar agent acts as a structure forming and stabilizing agent and has a very crucial role to play in the process of gelling. Water is the most commonly employed polar agent although some other polar solvents such as glycerol, ethylene glycol and form amide have also been found to possess the capability of transferring an initial non-viscous lecithin solution into jelly like state on organogel [22].
As described earlier, the major limitation in formation of lecithin organogels is the requirement of high purity lecithin, the high purity grade lecithin is not only expensive but also difficult to obtain in large quantities. However, recent reports indicates the incorporation of synthetic polymers i.e., pluoronic in lecithin organogels, for their usefulness as cosurfactant and stabilizer [32]. It has been shown that the inclusion of pluronic as cosurfactant makes the organogelling feasible with lecithin of relatively lesser purity [33]. The term “pluronic” refers to series of non-ionic closely related block copolymers of ethylene oxide and propylene oxide [29]. These are primarily used in pharmaceutical formulations as cosurfactants, emulsifier, solubilizers, suspending agents and stabilizers. These pluronic containing lecithin organogels have been termed as pluronic lecithin organogels, poloxamer organogels, pluronic organogels, PLO gel or simply PLOs.
Method of Preparation
The oil-surfactant mixture is heated at 60ºC to obtain a clear solution which on cooling forms organogels [34]. Based on the phase diagrams constructed, lecithin solutions are prepared by first dissolving lecithins in an organic solvents with the aid of magnetic stirrer. Formation of organogels takes place on addition of water with the help of micropiopette syringe. Sometime heat is applied for complete solubilization of drug [26].
The oil phase is prepared by mixing lecithin and organic solvent, the mixture is allowed to stand overnight to ensure complete dissolution. The aqueous (polar) phase is prepared by adding pluronic to ice cold water, the mixture is agitated to ensure complete dissolution. The prepared PLO, the oil phase is mixed with aqueous phase of pluronic using a high shear mixing method by magnetic stirrer [32].
Characterization of Organogels
In contrast to the ease of preparation, characterization of LOs is relatively complicated on account of their interior structural design build up on the selfassociated supramolecules. These microstructures, the resultant of varied polar non polar interactions, are highly sensitive and pose difficulties in the investigative studies. However, different characterization studies are extremely useful while investigating the potential applications of organogel systems as a topical vehicle. For instance, it has been reported that many of the physicochemical properties of Los viz. Rheological behavior, physical and mechanical stability, and drug release behavior are dependent upon how do molecules arrange themselves to provide the specific structural network within the organogel system [22, 35].
Phase-behavior of organogels For any vehicle to be used for topical drug delivery applications, it is essential to study its rheological behavior. The latter is important for it efficacy in delivering the molecules onto or across the skin site. The critical parameters like spreadibility, adhesiveness (property related to bioadhesion on skin site), cohesiveness (which indicates structural reformation following application of shear stress, and consistency need to be modified in a favorable manner. Lecithin organogels (LOs) have been studied extensively for their rheological attributes and determined to be viscoelastic in nature [22]. At higher lecithin concentrations, there is more extensive entanglement of long cylindrical micelles with each other, forming a network-like structure with a very high viscosity. The entrapment of the drug within this network lowers the amount of free drug available for release, causing a decrease in the release across the membrane [26]. Sameles containing different weight ratios (km) of lecithin/IPM (20:80) (40:60) (60:40) (80:20) are generally prepared, phase studies are carried out by adding water while stirring. After each addition of 1µ liter of aqueous phase of pure water to the lecithin solutions, the resulting systems are examined for clarity and viscosity. The course of each addition is monitored through cross polaroids in order to determine the boundaries of any organogel and briefringent liquid crystalline domains. The endpoint of the organogel domain at a given km is determined when the system became turbid after the addition of a specific amount of water. The phase behavior of the systems is mapped on phase diagrams with the top apex representing the lecithin and the other apices representing IPM and water solution. The transparent, homogeneous, nonbirefringent area enclosed by the line connecting the endpoints are considered as microemulsion based organogel [35].
Organogel structure and mechanism of organogelling The initially spherical reverse micelles that are formed by lecithin molecules in a nonpolar organic solvent transform into cylindrical micelles, once water is added. This is established with the help of light scattering and small angle neutron scattering techniques. This one dimensional growth of micelles is caused by the formation of hydrogen bonds between water molecules and phosphate groups of lecithin molecules so that two adjacent lecithin molecules are bridged together by one water molecule. IR and NMR spectroscopic methods have revealed that water molecules could interact simultaneously with phosphate groups of neighboring lipid molecules via hydrogen bonding, acting as a bridge between them. In this case solvent molecules and lecithin phosphate groups can arrange in such a way that a hydrogen-bonded network will be formed. The increase in the amount of water results in the formation of long tubular and flexible micelles. These micelles can be entangled and therefore build up a transient three-dimensional network, that is responsible for the viscoelastic properties of the lecithin organogels. At a critical concentration of water, network shrinks and phase separation occurs. At still higher contents of water a transformation to a solid, nontransparent precipitate can be observed. This diluted solution is composed of rodlike micelles, which their length is not enough to overlap and form a threedimensional network. The existence of microdomains of different polarity within the same single-phase solution enables water-soluble and oil-soluble drugs to be incorporated. This could be attributed either to the increase in the number of cylindrical micelles or to the further growth of the cylindrical micelles or both, leading to the increase in the solubilizing capacity [35].
Determination of gelation temperature
Formulations are enclosed in glass tubes (2 mm inside diameter) and observed over a temperature range of 4-5°C. The change from solution to gel or vice-versa are determined by inverting the tube. The temperature is changed at a rate of 5°C h? and the temperature at which the physical state of the formulation changes is regarded as the gelation temperature [36].
Gelation Kinetics The gelation properties of organogels are investigated in the presence of various solvents. Gel-sol and sol-gel transitions were evaluated by the inverse method and gelation kinetics are determined by turbidimetry [37].
In Vitro Drug Release The permeation apparatus designed as described by Chowdary et al. is employed to study the release profile of drugs from the semisolid formulations. The release/ permeation of drugs from lecithin gels through various membranes is determined using Franz diffusion cell [38].
Application and Future Prospects In the field of topical drug delivery, LOs have emerged as one of the most potential carrier systems. In contrast to other lipidbased system such as vesicular system (liposomes and niosomes) lecithinorganogel systems may prove to have an edge in term of efficacy, stability and most importantly, the technological feasibility. Moreover, the topical drug delivery of new biotech generated proteinaceous molecules in the protective non-polar microenvironment of these systems may help protect these sensitive macromolecules from and degradation, while their transport to the desired site. Thus, amidst the increasing opportunities and challenges, the LOs may prove to be highly promising system in realizing the drug delivery objectives while scientists are desperately trying for more viable alternative viz-a-viz existing carrier system. PLO is probably due to financial constrains as well as the industry focusing on area such as biotechnology and genomics. However, the great interest in PLO in the US has led to formulation of a second generation lecithin organogel premium, lecithin organogel base by Xenex Labs and Max Pharmaceuticals, USA. Table 1 shows some of the application and major findings of lecithin based organogels. A gel using hydroxy propyl cellulose and ethanol was formulated for transdermal delivery of testosterone. Testosterone loaded in the gel was 21 mg/cm2 [39]. Similarly, a hydrogel formulation of fentanyl or sufentanil was prepared using polyvinyl alcohol and resin buffer. The formulated gel had a skin contact area of 2 cm2 and 0.16 cm2 , respectively. The approximate weight of gel was 350 mg. This gel was delivered by electrortransport over 20 min in a dosage of 4 µg-5.5 µg [46]. The composition of transdermal formulation patented by Murdock et al. (2002) is summarized in Table 2. The workers extended this study to a combination of two active ingredients for the treatment of painful spasticity. Amitryptyline appeared to offer limited pain relief when administered transdermally. The results revealed that the combination of gabapentin with doxepine might offer additional benefit. The addition of guaifenesin to doxepine was proposed to be of particular value in cases of painful spasticity [47].
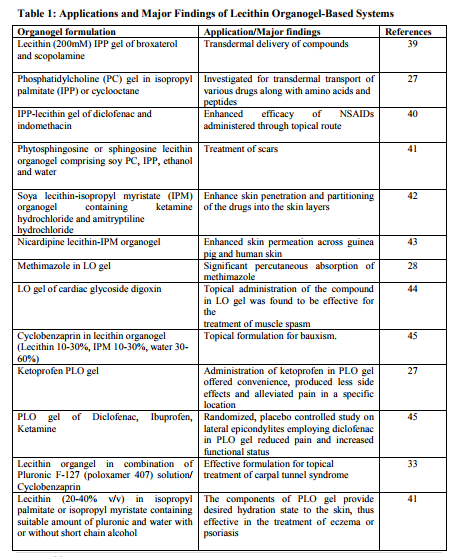
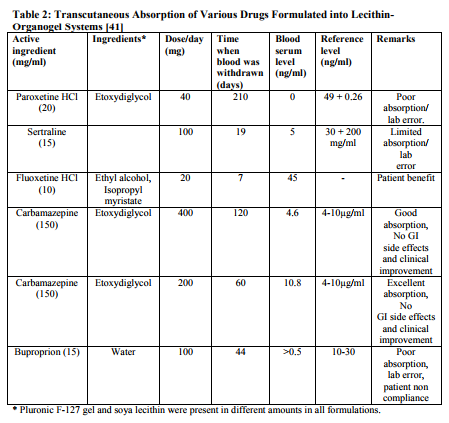
CONCLUSION
Lecithin, a biocompatible material has recently gained wide popularity for development of better drug delivery system. Interest in organogels has increased in a wide variety of fields including chemistry, biotechnology and pharmaceutics. Lecithin based delivery systems appear to be unique and industrially feasible approach to overcome the problem of low bioavailability associated with the lipophillic drugs. Lecithin based organogels as transdermal drug delivery provides the promising delivery system for lipophilic drugs. It provides better patient compliance by avoiding invasiveness, prolonging plasma drug level, bypass first pass effect, reduced side effects and easy termination of therapy.
References:
1. Prausnitz M R, Mitragotri S and Langer R (2004). Current status and future potential of transdermal drug delivery. Nat. Rev. Drug Dis, 3: 115- 24.
2. Nishihata T, Kotera K, Nakano Y and Yamazaki Y (1987). Rat percutaneous transport of diclofenac and influence of hydrogenated soya phospholipids. Chem. Pharm. Bull, 35: 3807-12. 31 International Journal of Current Research and Review www.ijcrr.com Vol. 03 issue 03 Mar 2011
3. Yokomizo Y (1996). Effects of phospholipids on percutaneous penetration of drugs through the dorsal of the guinea pig, in vitro.3.The effects of phospholipids on several drugs having different polarities. J. Control. Release, 42: 217-28.
4. Yokomiza, Y (1996). Effects of phophatidylcholine on the percutaneous penetration of drugs the dorsal skin of guinea pigs in vitro; and analysis of the molecular mechanism, using attenuated total reflectanceFourier Transform infrared (ATRFTIR) spectroscopy. J. Control. Release, 42: 249-62.
5. Kirjavainen M, Monkkonen J, Saukkosaari M, Valjakka koskela R, Kiesvaara J and Urtti A (1999). Phospholipids affect stratum corneum lipid bilayer fluidity and drug partitioning into the bilayers. J. Control. Release, 29: 207-14.
6. Touitou E, Dayan N, Bergelson L, Goodin B and Elias M (2000). Ethosomes-novel vesicular carriers for enhanced delivery: characterization and skin penetration properties J. Control. Release, 65: 403-18.
7. Vyas S P, Singh R and Asati R K (1995). Liposomally encapsulated diclofenac for sonophoresis induced systemic delivery. J. Microencapsul, 12: 149-54.
8. Woyczikowski B, Szuls J and Janicki S (1996). Effect of the mode of preparation of liposomes on trapping efficiency of sodium diclofenac. FarmPol. (Farmaja-polska), 52: 1011-5.
9. Dayan N and Touitou E (2000). Carriers for skin delivery of trihexyl phenidyl HCl: ethasomes vs liposomes. Biomaterials, 21: 1879-85.
10. Cevc G (1996). Transfersomes, liposomes and other lipid suspensions on the skin: permeation enhancement of vesicle penetration, and transdermal drug delivery. Crit. Rev. Ther. Drug Carrier Syst, 13: 257-388.
11. Scartazzini R and Luisi P L (1988). Organogels from lecithins. J. Phys. Chem, 92: 829-33.
12. Willimann H, Walde P, Luisi P L, Gazzaniga A and Stroppolo F (1992). Lecithin organogels as matrix for transdermal transport of drugs. J. Pharm. Sci, 81: 871-4.
13. Chowdary K P R and Madhavi B L R (2005). Novel drug delivery technologies for insoluble drugs. Indian Drugs, 42(9): 557-575.
14. Chowdary K P R and Vijayasrinivas S (2004). Biopharmaceutical classification system. The Indian Pharmacist, 111: 30, 7.
15. The Theory and Practice of Industrial Pharmacy. 3rd Edition by Leon L, Herbert AL and Joseph LK, Published by Varghese Publishing House, Bombay, P-221: 1987.
16. Tejal Shah (2005). Lipid emulsions as drug delivery systems – An overview. The Pharma Review, Jan-Feb: 41-48.
17. Sojan Jose and Kulkarni P K (2002). Self-emulsifying drug delivery system (SEDDS): Review. Indian J. Pharma. Educ, 36(4): 184-90.
18. Jayne Lawrence M, Gareth D and Rees (2000). Microemulsion-based media as novel drug delivery systems. Advanced Drug Delivery Reviews, 45: 89-121.
19. Tamilvanan S (2004). Oil-in-water lipid emulsion: implications for parenteral and ocular delivering systems. Progress in Lipid Research, 43: 489-533.
20. Aulton M E. Pharmaceutics: The Science of Dosage Form Design, 2nd 32 International Journal of Current Research and Review www.ijcrr.com Vol. 03 issue 03 Mar 2011 Edition, Published by Churchill Livingstone, London, P-503: 2002.
21. Kulkarni P K and Pradeep Kaatgi (2002). Emulsion-gels as topical drug delivery vehicle – A Review. Indian J. Pharm. Edu, 36(6): 119-123.
22. Kumar R and Katare O P (2005). Lecithin organogels as a potential phospholipids-structural system for topical drug delivery-A review. AAPS Pharma Sci. Tech. 4: 1-47.
23. Murdan S Gregoriadis G and Florescence A T (1999). Inverse toroidal vesicles: Precursor of tubules in sorbitan monostearate organogels. Inter. J. Pharma, 183: 47-49.
24. Lecithin organogels as model carrier of pharmaceutical; Edited by Zoumpanioti M, Karavas E, Skopelilis C published by Progress in Colloid and Polymer Science. Springer-Verlag Heidelberg, Vol. 123; 2004.
25. Scartazzini R and Loisi P C (1988). Organogels from lecithin. J. Phys. Chem, 92(3): 829-833.
26. Nasseri A A, Aboofazeli R, Zia H, Thomas E and Needham (2003). Lecithin-stabilized microemulsionsbased organogels for topical application of ketorolac tromethimine II. In vitro release study. Iran J. Pharm. Res, 5: 117-124.
27. William N, Walde P, Luisi L, Gazzaniga A and Stroppolo F (1992). Lecithin organogels as matrix for transdermal transport of drugs. J. Pharm. Sci, 81(9): 871-874.
28. Hoffmann G, Marks S L, Taboada J. Hosgood G L and Wolfsheiner K J (2003). Transdermal methimazole treatment in cats with hyperthyroidism. Journal of Feline medicine and surgery, 5: 27-82
. 29. Handbook of Pharmaceutical Excipients, edited by Ainley Wade and Paul J Waller. 2nd Edition, American Pharmaceutical Association, Washington published by Pharmaceutical Press, London, 257- 268, 352, 314, 316, 375: 1994.
30. Lecithin-organogels for topical delivery of drug. www.rx.ugr/ organogel _413htm
31. Valenta C, Wanka M and Heidlas J (2000). Evaluation of novel soyalecithin formulation for dermal use containing ketoprofen as a model drug. J. Cont. Release, 2(63): 165-173.
32. Murdan S (2005). A review of pluronic lecithin organogel as topical and transdermal drug delivery systems. Hospital Pharmacist, July-Aug: 267- 270.
33. Crandall W, Iso T. Topical moisturizing composition and method. US Paten 6 316 428; 2001.
34. Shelke V B, Thopte K, Wawde G M, Pisal S S and Kadam S S (2005). Nasal delivery of propranolol hydrochloride for sorbitan monostearate organogels: Preformulation study. Ind. J. Pharm. Sci, 67(2): 200-205.
35. Nasseri A A, Aboofazeli R, Zia H and Thomas E Needham (2003). Lecithinstabilized microemulsion: An organogel for topical application of ketorolac tromethamine I: Phase behaviour studies. Iran. J. Pharm. Res, 4: 120-124.
36. Bentley L B, Marchetti J M, Ricardo N, Ali Abi Z and Collett J H (1999). Influence of lecithin on some physicalchemical properties of ploxamer gels, rheological, microscopic and in vitro permeation studies. Int. J. Pharm, 193: 49-55.
37. Jean Christopher Leroux. In situforming pharmaceutical organogels based on the self assembly of C-alanine 33 International Journal of Current Research and Review www.ijcrr.com Vol. 03 issue 03 Mar 2011 derivatives. www.insitu forming/ organogels. Com.
38. Shrikande B K and Goupale D C (2001). Development and evaluation of anti-inflammatory oleogels of gurgul and curcumma longa, 38(120): 613- 616
. 39. Williamann H L and Luisi P L (1991). Lecithin organogels as matrix for the transdermal transport of drugs. Biochemical and Biophysical Research Communication, 177(3): 897-900.
40. Dreher F, Walde P, Walter P and Wehli E (1997). Interaction of a lecithin microemulsion gel with stratum corneum and its effect on transdermal transport. J. Control Release, 95: 131- 140.
41. Crandall, Wilson T. Method for topical treatment of scars with protein kinase C inhibitor. US Patent 6 306 2001.
42. Ford Peter R. Topical analgesic composition containing cream type carriers. US Patent. 2002028789, 2002.
43. Aboofazeli R Z, Hossein N and Thomas E N (2002). Transdermal delivery of nicardipine: An approach to in vitro permeation enhancement. Drug Delivery, 9(4): 239-247.
44. Charles C, Mallhew D. Cardiac glycosides for treating muscle pain and spasm. US Pat 20030229029, 2003.
45. Keith Anderson, Pharm P K Diffusimax-Transdermal-delivery, 1999 June. www.david.at.boomer.org.
46. Murdock, R W and Williams, C D. US2002647907B2, 2002.
47. Stinchcomb, A L and Swaan, P W. US20036569449B1, 2003.
|