IJCRR - 3(7), July, 2011
Pages: 37-51
Print Article
Download XML Download PDF
EFFECT OF SOLANUM TRILOBATUM EXTRACT ON ERYTHROCYTE MEMBRANE SURFACE CHARGE
IN AGED RATS
Author: Amarnath Kanchana , Chinnakannu Panneerselavam
Category: Healthcare
Abstract:Objectives: Oxidative stress an unavoidable consequence of oxygen metabolism in aerobic cells is postulated to be one of the most important causes of age related changes. The changes induced by free radicals are believed to be the major source of ageing, disease development, destruction of normal cell function and membrane rigidity. The present study was intended to determine the effect of chloroform extract of Solanum trilobatum (CST) on membrane surface charge density in aged rat erythrocytes upon oxidative stress. Methods: The leaves of Solanum trilobatum were subjected to chloroform and the extract was prepared. Chloroform extract of Solanum trilobatum (CST) (150 mg/day/kg body weight) was administered orally
for 90 days to young and aged rats. The erythrocyte membrane was isolated and was used to perform the
studies such as estimation of protein carbonyls, glycoproteins, surface charge and enzymatic and non
enzymatic levels. Key findings: A noteworthy decline in surface charge levels with simultaneous increase in protein carbonyls and shrink in glycoprotein and antioxidants status was prominent in erythrocytes of aged rats contrast to young rats. Administration of CST improved the erythrocyte surface charge density to near normalcy in aged rats. In addition a decrease in protein carbonyls level and increase in glycoproteins as well as antioxidant status was observed in aged rat erythrocytes on CST therapy.
Conclusions: Therefore the polyphenols and flavanoids are the phytochemical compounds in CST that might play a central role in defending the oxidative stress related loss of membrane surface charge in a way maintaining the erythrocyte membrane integrity and functions in aged individuals.
Keywords: Aging; Erythrocyte surface charge; Protein carbonyls; Glycoprotein; Antioxidants; Solanum trilobatum; Phytochemicals.
Full Text:
INTRODUCTION
Oxidative damage apparently increases with age and thus may overwhelm the natural repair system in the organism1 leading to the onset of age-related diseases with a progressive increase in the chance of morbidity and mortality. At the cellular level, the reactive oxygen species inclination due to oxidative stress damages vital cell components like poly unsaturated fatty acids, protein and nucleic acids2 . Free radical attack on cellular proteins, damages the proteins3 leading to oxidation of side chains of lysine, arginine, proline and threonine thereby yield protein carbonyl derivatives the markers of protein oxidation4 . These oxidative modifications cause loss of structural and catalytic functions of the cell contributing to serious deleterious changes affecting cell survival5 . The cell membrane is a structural barrier that plays an essential role in protecting cellular integrity and underlying cause of ageing process6 . Changes in the macromolecules of membrane are one of the earliest signs of erythrocytes membrane alterations during ageing. Reactive oxygen species generated in the aqueous or lipid phase can attach to the erythrocyte membrane and can induce oxidation of lipids, proteins and carbohydrates, triggering disruption in membrane7 and further enhances the development of one or better-recognized age related modifications, such as alteration of intrinsic membrane properties (fluidity, ion transport, loss of enzyme activity, protein crosslinking), inhibition of protein synthesis and DNA damage4 , ultimately resulting in the cell death. Carbohydrates in erythrocytes have many important functions that are necessary for the proper functioning of the cell to survival. Carbohydrate is thought to be highly necessary for cell surface negative charges and have a crucial role in the clearance of senescent cells and essentially to prevent aggregation of erythrocytes from each other8 . Like most biological surfaces, erythrocytes exhibit a negative surface charge that is mainly attributed to sialic acid residues located on the glycoproteins in the membrane surface9 . Oxidative stress or other damaging effects to surface sialosaccharide may itself play role in aggregation of erythrocytes, increasing the adhesiveness to endothelial cells contributing for the development of various pathologies including diabetes mellitus, atherothrombotic complications10and sequestration of circulating erythrocytes by macrophages11 . Age associated oxidative changes in the glycomoieties of erythrocyte glycoprotein affects the surface charge including aggregation and binding of erythrocytes to macrophages12 and found to be associated with cardiovascular risk factors such as, hypertension, hyperlipoproteinemia and myocardial ischemia13and thus crucial for erythrocyte survival14 . During lifetime, an antioxidant network counteracts the deleterious action of free radicals and reactive species on macromolecules. Cells synthesize some of their antioxidant enzymes, as the superoxide dismutase, catalase, and glutathione peroxidase, as well as the peptides with thiol groups, as glutathione, while other non-enzymatic antioxidants from nature through nutrition, as vitamin C, vitamin E, and carotenoids. Several repair systems help antioxidant action by the recovery of damaged macromolecules15. Together, these systems play an important role in the ability of the body to respond to the oxidant challenge of using molecular oxygen to drive reactions that yield the necessary energy for life processes. However various anti ageing agents are being ushered into the field of medicine to conflict ageing and age-associated disorders. Flavonoids, the potential dietary antioxidant found ubiquitously in plants, attracted global interest in combating the devasting effects of oxidation in cells and tissues of an organism. Dietary antioxidants are considered beneficial because of their potential protective role against oxidative stress, which is involved in the pathogenesis of multiple diseases such as cancer and coronary heart disease16,17. The potential antioxidant effect in vivo of individual food polyphenols (PP) or concentrated extracts has been widely investigated in cultured cells18,19 live animals20 and men21,22,23. Herbal remedies as antioxidant supplement is thus globally growing up owing to broad spectrum of beneficial biological properties. Owing to the presence of phenolic compounds and the free radical scavenging properties24 of an anti cancer herabal extract Solanum trilobatum25 , the present study is carried out to study the effect of Chloroform extract of Solanum trilobatum (CST) on erythrocyte membrane surface charges during animal aging. The study also explores the effect of CST on erythrocyte membrane protein carbonyl levels and the antioxidant status in aged rats.
2. MATERIALS AND METHODS
2.1. Chemicals
Dextran 500 (500,000 molecular weight), Polyethylene glycol (PEG, 8000 molecular weight), Bovine serum albumin (BSA), and 2,4- dinitro-phenylhydrazine (DNPH) were purchased from Sigma Chemical and Company, Saint Louis, MO, USA. All other chemicals were of reagent grade .
2.2. Preparation of Chloroform extract of Solanum trilobatum (CST)
Extraction of plant materials
The leaves of Solanum trilobatum were collected from the local market and samples of the plant were identified and authenticated by Dr. Brindha, Botanist, Dept. of Pharmacognosy, Captain Srinivasa Murti Drug Research Institute for Ayurveda (CCRAS, New Delhi), Arumbakkam, Chennai, Tamil Nadu. The fresh leaves were shad dried, powdered and extracted successively with 1.2 L of chloroform, in a Soxhlet extractor for 18-20 h. The extracts were concentrated to dryness under reduced pressure and controlled temperature (40o -50oC). The chloroform, extract yielded a brown semisolid residue weighing 6.2 g (2.39% w/w). The extract was then filtered through Whatmann No.1 filter paper and concentrated using concentrator. Then it was frozen and subjected to lyophilization .
2.3. Animals, treatment and fixation of dosage and duration
Male albino rats of Wistar strain, weighing approximately 120–160 g (young) and 380–420 g (old) were used. The animals were obtained from King Institute of Preventive Medicine, Chennai. The animals were divided into two major groups namely, Group Ia: normal young rats (3–4 months old) and Group IIa: normal aged rats (about 24 months old) and two experimental group Id (Young rats) and group IId (Aged rats) on CST administration for 90 days. Each group consisted of six animals. The animals were maintained on commercial rat feed containing 5% fat, 21% protein, 55% nitrogen free extract, 4% fiber (w/w) with adequate mineral and vitamin contents and had access to water ad libitum. CST (Chloroform extract of Solanum trilobatum) was supplemented orally (oral gavage) at the dosage of 150 mg/kg body weight/day for 90 days, whereas young control and aged control rats received vehicle alone in a similar manner. On completion of 90 days of CST supplementation, the blood was collected with 3.8% sodium citrate from jugular vein and used for the isolation of erythrocytes and erythrocyte membranes. Fixation of dose and duration: Trial studies were carried out with different concentrations of Chloroform extract of Solanum trilobatum (50, 100, 150, 200 and 250 mg) dissolved in physiological saline and supplemented at various durations (30, 60, 90 and 120 days) orally to rats. The effectual dosage (150 mg/kg body weight/day) was selected on the basis of the concentration at which Chloroform extract of Solanum trilobatum was capable of inhibiting lipid peroxidation significantly above which there was (200 and 250 mg/kg body weight) no significant inhibition of lipid peroxidation. Similarly the effectual duration was found to be 90 days above which there was insignificant inhibition of lipid peroxidation. As per the results obtained, the forthcoming biochemical and molecular parameters were carried out with Chloroform extract of Solanum trilobatum supplementation for 90 days alone. The body weight of the animals were increased at the end of experiment as in group Ia (from 74.50 ± 5.6 to 80.40 ± 6.7), group Id (from 74.50 ± 5.6 to78.30 ± 3.3), group IIa (from 120.4 ± 4.8 to 155.9 ± 5.0) and group IId (from 126.2 ± 5.0 to 162.0 ± 5.3) in respect to their initial body weight.
2.4. Preparation of erythrocytes and erythrocyte membranes
Isolation of erythrocytes and erythrocyte membranes was done according to Dodge et al. (1963)26 with slight modifications. Briefly, blood collected from animals with 3.8% sodium citrate was subjected to centrifugation at 3000 rpm for 10 min at 4 0C. The plasma and buffy coat were removed by aspiration and the erythrocytes were washed three times with cold (4 0C) phosphate-buffered saline (PBS: 0.15 M NaCl, 1.9 mM NaH2PO4, 8.1 Mm Na2HPO4, pH 7.4). Washed erythrocytes were hemolyzed in 40 volumes of 5 mM sodium phosphate buffer (pH 8.0) (containing 1 mM EDTA and 0.5 mM phenylmethylsulfonyl fluoride, as protein inhibitor), and centrifuged for 20 min at 4 0C at 15,000 rpm. The supernatant (or hemolysate) was decanted carefully and saved, while the pellet were washed repeatedly and incubated for 45 min at 370C to reseal the membrane in the presence of 0.8 Mm MgCl2-ATP solutions, for hemoglobin free ghosts. Hemoglobin amount was estimated by Drabkin and Austin (1932)27 method and erythrocyte membrane protein content by Lowry et al. (1951)28 .
2.5. Determination of erythrocyte surface charge
Erythrocyte partition coefficients were determined at room temperature by a two-phase aqueous system containing 5% Dextran 500 and 4.3% PEG 8000 in isotonic phosphate buffer (pH 6.8) by the method of Walter (1985)29 . The two-phase system used is ?charge sensitive‘ whose partition coefficient ratio indicates the level of erythrocyte surface charge.
2.6. Determination of protein carbonyls
Protein carbonyls content was determined by the reliable method based on the reaction of carbonyl groups with 2, 4- dinitrophenylhydrazine to form 2, 4-dinitrophenylhydrazone as suggested by Levine et al. (1994)30 .
2.7. Determination of glycoproteins
Lipids were extracted from the erythrocyte membrane pellets according to the method of Folch et al. (1957)31using chloroform–methanol mixture (2:1 v/v). The resulting defatted residue was suspended in sodium acetate buffer (containing 2 mg cysteine HCl/ml, final pH 7.0) and deproteinized by 4–5 volumes of ethanol, evaporated to dryness in the cold under a vacuum and subjected to hydrolysis by heating with 2 ml of 4 N HCl for 4–6 h. The hydrolyzed material was neutralized with 4 N sodium hydroxide and used for estimating erythrocyte hexose (Niebes, 1972)32, hexosamine (Wagner, 1979)33 and sialic acid (Warren, 1959)34 .
2.8. Determination of antioxidants
Superoxide dismutase (SOD) in hemolysate was assayed by the method of Marklund and Marklund (1974)35, while erythrocyte membranes were used to estimate catalase (CAT) (Beers and Seizer, 1952)36. Hemolysate glutathione peroxidase (GPx) was determined by Rotruck et al. (1973)37 method, glutathione reductase (GR) by the procedure of Staal et al. (1969)38, glucose-6-phosphate dehydrogenase (G6PD) by Zinkham et al. (1958)39 and glutathione-S-transferase (GST) according to Habig et al. (1974)40. Hemolysate reduced glutathione (GSH) was estimated by Moron et al. (1979)41 and oxidized glutathione (GSSG) was assayed according to the method of Tietze (1969)42. Erythrocyte redox state was determined by the redox index: (GSH + 2 X GSSG) / (2 X GSSG / 100).
2.9. Statistical analysis
The results are expressed as mean standard deviation (SD) for six rats in each group. Differences between groups were assessed by one-way ANOVA using the SPSS version 7.5 software packages for Windows, USA. Post hoc testing was performed for inter-group comparisons using the least significance difference (LSD) test; statistical significance at p-values<0.05, have been given respective symbols in the figures and tables.
. RESULTS
Figure 1 illustrates surface charge in erythrocytes of young and experimental rats in terms of partition coefficient ratio. Significant (p < 0.05) decrease in surface charge level in erythrocytes was noticed in aged rats when compared to young rats. CST supplementation to aged rats showed increment in surface charge (p<0.05) when compared to control aged rats. The result illustrated significant surface charge loss and increased free radical attack on membrane proteins in aged animal. Figure 2 shows age related changes in protein carbonyls in erythrocytes of control and Chloroform extract of Solanum trilobatum treated young and aged rats. Increase in protein carbonyl by 1.7 fold was observed in erythrocyte membrane and plasma of aged rats when compared with young rats. Such an elevation in protein carbonyl content indicates increased protein oxidation with advancement of animal age. Chloroform extract of Solanum trilobatum treatment to aged rats showed significant decrease in protein carbonyl levels by 1.6 fold in erythrocytes and 1.5 fold in plasma. The observed partition coefficient ratio and protein carbonyls level were normal in erythrocytes of control and Chloroform extract of Solanum trilobatum treated young rats. Thus the results reveal the membrane integrity and functions are maintained at normal levels in erythrocytes of young rat. Figure 3 depicts the levels of glycoproteins like hexose, hexosamine and sialic acid in erythrocyte membrane of control and Chloroform extract of Solanum trilobatum treated young and aged rats. The glycoprotein levels in erythrocytes of young rats were at normal level and did not show any significant alterations on Chloroform extract of Solanum trilobatum treatment. Profound decrease in the levels of hexose, hexosamine and sialic acid by 23%, 33% and 22 % respectively in erythrocytes of aged rats was observed. However supplementation of Chloroform extract of Solanum trilobatum enhanced the level of hexose by 30%, hexosamine by 40% and sialic acid by 23% in aged rat erythrocytes. Figure 4 highlights the activities of enzymatic antioxidants SOD, CAT, GPx, GST, GR and G6PD in erythrocytes of young and aged rats. Young control rats had normal level of enzymatic antioxidants and showed insignificant changes on CST supplementation. The activities of SOD, CAT GPx, GR, GST and G6PD were decreased significantly by 33%, 47% 38% and 39% 39% and 45%respectively in aged rats when compared to young rats. However CST supplementation to aged rats improved the activities of SOD by 31%, CAT by 68%. GPx by 39%, GST by 43%, GR by 44% and G6PD by 59%. Table 1 demonstrates the glutathione status in erythrocytes of control and CST treated young and aged rats. Erythrocyte glutathione status was observed to be at normal levels in control and treated young rats. Remarkable decline in GSH level (38%) with increase in GSSG level (66%) was noted in aged rat erythrocytes. These alterations indicate increased oxidation of glutathione in erythrocytes with advancement of age. Further significant decrease in both GSH/GSSG ratio and redox index by 2.7 fold was also observed in aged rat erythrocytes. CST treatment to aged rats increased the GSH level by 41% and reduced GSSG levels by 40% with subsequent increase in GSH/GSSG ratio by 2.3 fold and redox index by 1.4 fold.
4. DISCUSSION
Cell membrane is an important target for all radical damages and blood can reflect the liability of the whole animal to oxidative conditions, erythrocytes and erythrocyte membranes have been used extensively for determining the effects of ageing. Change in erythrocyte membrane accompanying red cell ageing, lead to certain disorders such as - thalasemia, sickle cell anemia, and hereditary spherocytosis and therefore responsible for the selective removal of erythrocytes prematurely from circulation. Preservation of the cell membrane structure and suitable charge levels on its surface decides the accurate course of many processes. Alteration in erythrocyte membrane surface charge being a measure of cell condition indicated the augmented risk of erythrocytes aggregation involving in the changes in erythrocyte structure, functions and pathologic conditions such as chronic renal insufficiency and purulent intoxication43. In accordance the present study demonstrated a significant reduction of surface charge in aged rats when compared with young rats. This decrease could be possibly due to the increased protein oxidation leading to carbonyl formations in aged rat erythrocytes. Consequently, our results are in accordance with the work of Sangeetha et al., 200514 who also specifies modifications in the surface charge due to an increase in the protein carbonyl levels in aged rat erythrocytes. Furthermore, enhanced level of carbonyl formation can be endorsed to age dependent changes in the rate of oxidized protein degradation44, 45. Addition reactions by highly reactive intermediate products of LPO or and glycosidation, and direct oxidative modification of macromolecules during oxidative stress may probably be the reasons for the modification of proteins46. Though several antioxidant defense systems have evolved to prevent free radical mediated damage, oxidized proteins appear to accumulate with animal age47 and may represent 30-50% of the total protein in old cells48 . Thus, increased oxidative damage on membrane proteins during animal aging may lead to the attenuation of negative surface charge in erythrocytes. Additionally, the observed decrease in glycoproteins may also be attributed to the surface charge determination with advancement of animal age. It has been publicized that sialosaccharide chains of glycophorin A changes the sialic acid levels upon oxidation that eventually decreases membrane surface charges thereby contributing to rouleaux formation11 . Earlier studies have made known, decline in sialic acid levels on erythrocytes decreases surface charge level leading to the appearance of non-IgG covered epitopes on the surface of oxidized erythrocytes49, therefore allowing the recognition of erythrocytes by macrophages11 . Furthermore, Vomel (1984)50 has confirmed the decrease of sialic acid in old individuals result in surface charge modifications. Chloroform extract of Solanum trilobatum supplementation lead to an increase in glycoproteins especially sialic acid level and ultimately increased the membrane surface charge. A significant increase in surface charge noted in erythrocytes of Chloroform extract of Solanum trilobatum treated rats would have been possibly due to the preservation of protein carbonyl levels by polyphenolic compounds functioning as an in vivo antioxidant that prevents protein oxidation51 with advancement of age. These alterations may perhaps be due to the free radical scavenging and reduced thiol group elevating properties of phytochemicals52that protects the membrane proteins from oxidative insults. Moreover the ability to maintain redox state of sulphydryl groups in membrane proteins by flavonoids53would has contributed to the maintenance of glycoprotein levels and thus the membrane surface charge in erythrocytes of aged rats. The inequity flanked by protective antioxidant defense and increased radical production during aging would transform the red cells towards oxidative stress resulting in amendment of membrane properties and cell dysfunction. The biological effects of reactive oxygen species are tightly controlled by a wide spectrum of enzymatic antioxidant defense systems54 . Erythrocytes being regularly exposed to free radicals are however equipped with antioxidants far in excess of normal requirement and also function as an effective oxidative sink in the organism55 . Enzymatic antioxidants present in the cells significantly delay and prevent oxidation and related damages. Cu-Zn SOD, a major antioxidant enzyme in erythrocytes, protects against oxygen free radicals by catalyzing the removal of superoxide radical (O2 - ) and catalase, (CAT) a haemoprotein, primarily works to catalyze the decomposition of hydrogen peroxide to water. In particular, the oxidation or autooxidation of hemoglobin (Hb- - the continuous formation of superoxide radicals 56 which is reflected as declined activities of SOD and CAT in erythrocytes of aged rat in the present study. Because cellular membranes house the production apparatus of these radicals and since membranes suffer great damage from these radicals, modification of macromolecules has been proposed to play a major role in the process of animal ageing57. Significant increase in SOD and CAT activity on supplementation of Chloroform extract of Solanum trilobatum to near normalcy in aged rat erythrocyte may be due to the potential quenching of free radicals24 by phenolic acids present in it. Further reports have suggested that polyphenols in CST are effectual scavenger of superoxide and hydroxyl radicals58 thereby sparing the antioxidant enzymes valuable in protecting erythrocytes from oxidative damages. Glutathione, a tripeptide containing -glutamic acid, cysteine and glycine, provides the first line of defense against ROS and protects erythrocytes from oxidative damage that acts as a ?radical quencher?. The glutathione redox enzymes, glutathione peroxidase, glutathione-stransferase, glutathione reductase and glucose-6- phosphate dehydrogenase provide the second line of defense through detoxification of noxious by-products and alleviation of free radicals mediated macromolecular damages in erythrocytes59 . An intense increase in the level of GSSG with a concomitant depletion in the concentration of GSH, the redox status and the glutathione metabolizing enzymes may represent an imbalance in prooxidant and antioxidant status in aged rats. Significant decrease in the activity of glutathione metabolizing enzymes is well correlated with the declined availability of its substrate, glutathione with advancement of age14. The elevated exposure of erythrocytes to free radicals may likely be the motivation for GSH depletion6 . Further studies suggested that enhanced utilisation of glutathione by enzymes such as GPx, GST and reduced activity of glutathione regenerating enzyme G6PD, due to the upsurge of reactive oxygen species production may decrease the GSH status in erythrocytes of aged animals61. Thus fall in the activity of G6PD essential for an adequeate supply of NADPH (enzymes involved in GSH regeneration) creates an imbalance in GSH/GSSG ratio62 and thereby a shift in the redox state of cells with advancement of age63 . The flavanoids and polyphenols in Chloroform extract of Solanum trilobatum protected the sulphydryl groups and thiols from oxidative damage thereby elevating their levels64. Also the metal chelating action of Chloroform extract of Solanum trilobatum improved the activities of glutathione metabolizing enzymes (GPx, GST, GR, and G6PD) in aged rats65 . Moreover the decrease in free radical levels by polyphenols in CST might also have contributed to the improvement of glutathione metabolizing enzymes66 .
5. CONCLUSION
Ageing can thus be viewed as an irreversible process associated with the accumulation of these oxidative changes wherein Chloroform extract of Solanum trilobatum potentially enhances the antioxidants levels by protecting erythrocyte membrane from free radical attack and eventually preventing the loss of surface charge levels during ageing. The outcome of the present study thus indicates, Chloroform extract of Solanum trilobatum supplementation could be an exact therapy for minimizing the age related alterations in erythrocytes for the existing population, as the health care and welfare of the aged have been attracting considerably as a social problem.
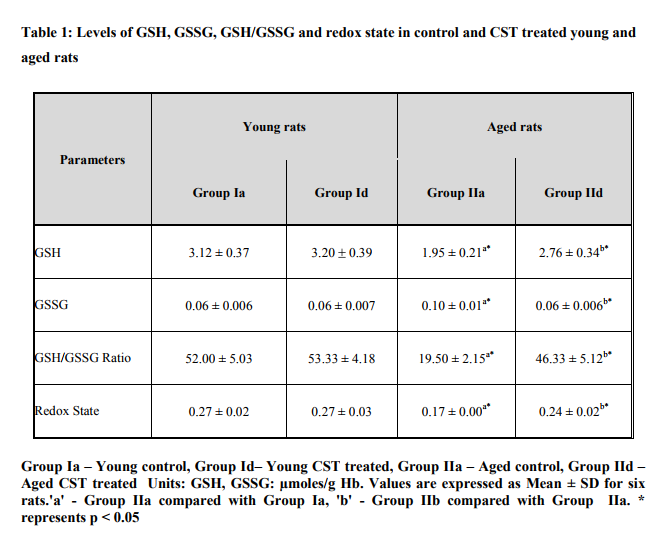
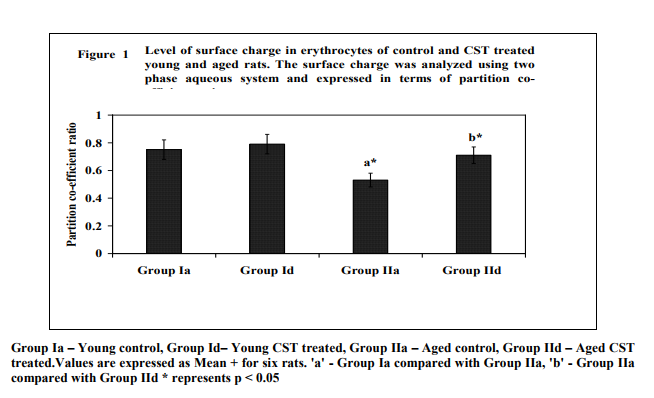
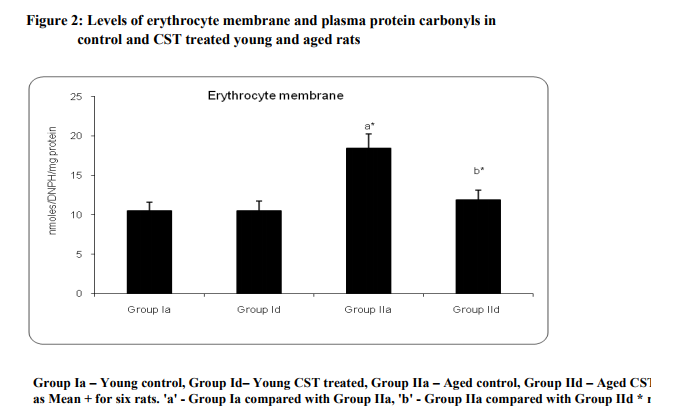
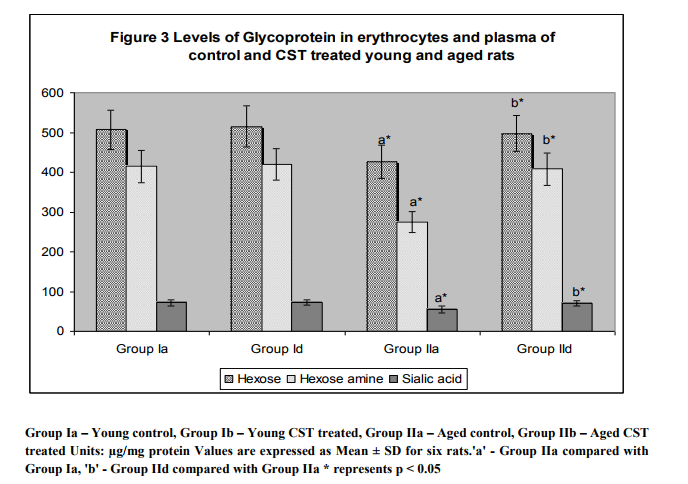
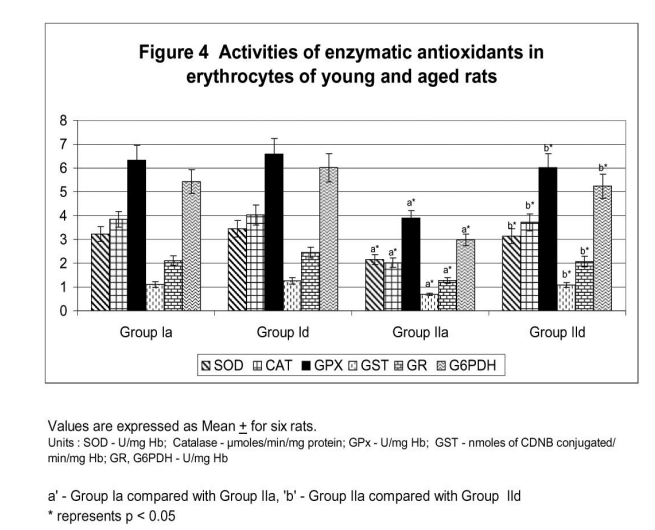
References:
1. Kowald A. Theoretical Gompertzian implications on life span variability among genotypically identical animals. Mech Ageing Dev. 1999; 110:101-7.
2. Halliwell B, The antioxidant paradox. Lancet 2000; 355:1179 – 1180.
3. Sinclair AJ, Barnett AH, Lunec J. Free radicals and antioxidant systems in health and disease. Br J Hosp Med. 1990; 43: 334- 44.
4. Bandyopadhyay U, Das D, Banerjee RK. Reactive oxygen species, oxidative stress and pathogenesis. Curr. Sci. 1999; 77:658– 666.
5. Levine RL, Williams JA, Stadtman ER, Shacter E. Carbonyl assays for determination of oxidatively modified proteins. Methods Enzymol. 1994; 233: 346-57.
6. Spitelle,r G. Are changes of the cell membrane structure causally involved in the aging process? Ann N Y Acad Sci. 2002; 959:30-44.
7. Grune T, Shringarpure R, Sitte N, Davies K. Age-related changes in protein oxidation and proteolysis in mammalian cells. J Gerontol A Biol Sci Med Sci. 2001; 11:459-67.
8. Jovtchev S, Djenev I, Stoeff S, Stoylov S. Role of electrical and mechanical properties of red blood cells for their aggregation. Colloids Surf. Physiochem. 2000; 164:95–104.
9. Aminoff D. Methods for the quantitative estimation of N-acetylneuraminic acid and their application to hydrolysates of sialomucoids.. Biochem J. 1961; 81:384- 92.
10. Wautier JL, Paton RC, Wautier MP, Pintigny D, Abadie E, Passa P, Caen J. Increased adhesion of erythrocytes to endothelial cells in diabetes mellitus and its relation to vascular complications. N Engl J Med. 1981; 305: 237-42.
11. Beppu M, Hayashi T, Hasegawa T, Kikugawa K. Recognition of sialosaccharide chain of glycophorin on damaged erythrocytes by macrophage scavenger receptors. Biochim. Biophys. Acta. 1995; 1268: 9-19.
12. Ando K, Kikugawa K, Beppu M. Binding of anti-band 3 autoantibody to sialylated poly-N-acetyllactosaminyl sugar chains of band 3 glycoprotein on polyvinylidene difluoride membrane and sepharose gel: further evidence for anti-band 3 autoantibody binding to the sugar chains of oxidized and senescent erythrocytes. J Biochem. 1996; 119:639-47.
13. Chien S, Weinburg R, Li S, Li Y. Endobeta-N-acetylglucosaminidase from fig latex. Biochem Biophys Res Commun. 1976; 76:317-23.
14. Sangeetha P, Balu M, Haripriya D, Panneerselvam C. Age associated changes in erythrocyte membrane surface charge: Modulatory role of grape seed proanthocyanidins. Exp Gerontol. 2005; 40: 820-8
. 15. Cutler RG, Rodriguez H. (Eds.). Critical Reviews of Oxidative Stress and Aging: Advances in Basic Science, Diagnostics and Intervention. World Scientific Publishing, Singapore. 2003; 1523.
16. Duthie GG, Duthie SJ, Kyle JAM. Plant polyphenols in cancer and heart disease: implications as nutritional antioxidants. Nutr Res Rev. 2000; 13:79–106.
17. Trichopoulou A, Vasilopoulou E. Mediterranean diet and longevity. Br J Nutr. 2000; 84:205–9.
18. Youdim KA, Martin A, Joseph JA. Incorporation of the elderberry anthocyanins by endothelial cells increases protection against oxidative stress. Free Rad Biol Med. 2000; 29:51–60.
19. Schroeter H, Spencer JPE. Rice-Evans C, Williams RJ. Flavonoids protect neurons from oxidized lowdensity-lipoproteininduced apoptosis involving c-Jun Nterminal kinase (JNK), c-Jun and caspase- 3. Biochem J. 2001; 358:547–57.
20. Hininger JF, Dragst LO, Daneshvar B, Lauridsen ST, Hansen, Sandstrom MB. The effect of grape-skin extract on oxidative status. Br J Nutr. 2000; 84:505–13.
21. Abu-Amsha Caccetta R, Burke V, Mori TA, Beilin LJ, Puddey IB, Croft KD. Red wine polyphenols, in the absence of alcohol, reduce lipid peroxidative stress in smoking subjects. Free Rad Biol Med. 2001; 30:636–42.
22. Natella F, Ghiselli A, Guidi A, Ursini F, Scaccini C. Red wine mitigates the postprandial increase of LDL susceptibility to oxidation. Free Rad Biol Med. 2001; 30:1036–44.
23. Breinholt V, Lauridsen ST, Dragsted LO. Differential effects of dietary flavonoids on drug metabolizing and antioxidant enzymes in female rat. Xenobiotica. 1999; 29:1227– 40.
24. Monavalli B, Raja Rajeswari A, Gowri V, Kanchana A. Invitro Antioxidant Activity of Methanolic Extract of Solanum trilobatum Leaves. J. of Natural Science and Technology - Life Sciences and Bioinformatics. 2010; 2:168-174.
25. Mohanan PV, Devi KS. Effect of Sobatum on radiation-induced toxicity in mice. Cancer Lett. 1998; 123:141-5.
26. Dodge JT, Mitchell C, Hanahan DJ. The preparation and chemical characteristics of hemoglobin-free ghosts of human erythrocyte. Arch. Biochem. Biophys. 1963; 100:119–130.
27. Drabkin DL, Austin JH. Spectrophotometric studies, spectrophotometric constants for common hemoglobin derivatives in human, dog and rabbit blood. J. Biol. Chem. 1932; 98:719– 733.
28. Walter H. Surface properties of cells reflected by partitioning red blood cells as a model. In: Walter H, Brooks, D.E., Fisher, D. (Eds.), Partitioning in Aqueous TwoPhase Systems. Academic, Orlando, FL, 1985; 327–376.
29. Lowry OH, Rosebrough NJ, Farr AL, Randall RJ. Protein measurement with the Folin phenol reagent. J. Biol. Chem. 1951; 193:265– 275.
30. Levine RL, Williams JA, Stadtman ER, and Shacter E. Carbonyl assay for determination of oxidatively modified proteins. Methods Enzymol. 1994; 233:346—357.
31. Folch J, Less M, Sloane Stanley GH. A simple method for the isolation and purification of total lipids from animal tissues. J. Biol. Chem. 1957; 226:497–509.
32. Niebes P. Determination of enzymes and degradation products of glycosaminoglycans metabolism in the serum of healthy and varicose subjects. Clin. Chim. Acta. 1972; 42:399–408.
33. Wagner WD. A more sensitive assay discriminating galactosamine and glucosamine in mixtures. Anal. Biochem. 1979; 94:394–396.
34. Warren L. The thiobarbituric acid assay of sialic acid. J. Biol. Chem. 1959; 234:1971– 1975.
35. Marklund S, Marklund G. Involvement of the superoxide anion radical in the autooxidation of pyrogallol and a convenient assay for superoxide dismutase. Eur. J. Biochem. 1974; 47:469–474.
36. Beers RF, Seizer IW. A spectrophotometric method for measuring breakdown of hydrogen peroxide by catalase. J. Biol. Chem. 1952; 195:133–140.
37. Rotruck JT, Pope AL, Ganther HE, Swanson AB, Hafeman DG, Hoekstra WG,. Selenium: biochemical role as a component of glutathione peroxidase purification and assay. Science 1973; 179:588–590.
38. Staal GE, Visser J, Veeger C. Purification and properties of glutathione reductase of human erythrocytes. Biochim. Biophys. Acta 1969; 185:39–48.
39. Moron MS, Depierre JW, Mannervik B. Levels of glutathione, glutathione reductase and glutathione S-transferase activities in rat lung and liver. Biochim. Biophys. Acta. 1979; 582:67–78.
40. Habig WH, Pabst MJ, Jakoby WB. Glutathione S-transferases, the first enzymatic step in mercapturic acid formation. J. Biol. Chem. 1974; 249:7130– 7139.
41. Zinkham WH, Lenhard RE, Childs B. A deficiency of glucose-6-phosphate dehydrogenase activity in erythrocytes from patients with favism. Bull. Johns Hopkins Hosp. 1958; 102:169–175.
42. Tietze F. Enzymic method for quantitative determination of nanogram amounts of total and oxidized glutathione: applications to mammalian blood and other tissues. Anal. Biochem. 1969; 27:502–522.
43. Samoilov MV, Mishnev OD, Kudriavtser IV, Naumor AG, Daniltov AP. Morphofunctional characteristics of erythrocytes in chronic kidney failure and purulent intoxication. Klin. Lab. Diagn. 2002; 6:18–23.
44. Stadtman ER. Protein oxidation in aging and age-related diseases. Ann N Y Acad Sci. 2001; 928, 22-38
45. Beal MF. Oxidatively modified proteins in aging and disease. Free Radic Biol Med. 2002; 32: 797-803.
46. Hyun DH, Lee M, Halliwell B, Jenner P. Proteasomal inhibition causes the formation of protein aggregates containinga wide range of proteins, including nitrated proteins. J Neurochem. 2003; 86:363-73
47. Arivazhagan P, Panneerselvam C. Favorable effect of L-Carnitine supplementation on glycoprotein status in aged rats. J. Clin. Biochem. Nutr. 1999; 26:193–200.
48. Carney JM, Starke-Reed PE, Oliver CN, Landum RW, Chen MS, Wu JF, Froemming GR, O‘Brien NM. U937 cells as a model to study the effect of phytochemicals on superoxide anion production. Nutr Res. 1997; 17:1091–1103.
49. Leb L, Snyder LM, Fortier NL, Anderson M. Antiglobulin serum mediated phagocytosis of normal senescent and oxidized RBC: role of anti-IgM immunoglobulins in phagocytic recognition. Br. J. Haematol. 1987; 66:565–570.
50. Vomel T. Properties of ATPases and energy-rich phosphate in erythrocyte of young and old individuals. Gerontology. 1984; 30:22–25.
51. Sato M, Maulik N, Das DK. Cardioprotection with alcohol: role of both alcohol and polyphenolic antioxidants. Ann N Y Acad Sci. 2002; 957:122-35.
52. Kalyan Reddy, Manda Craig Adams, Nuren Ercal. Biologically important thiols in aqueous extracts of spices and evaluation of their in vitro antioxidant properties. Food Chemistry. 2010; 118:589-593.
53. VanDuijn MM, Van den Zee J, Vansteveninck J, Van den Broek PJ. Ascorbate stimulates ferricyanide reduction in HL-60 cells through a mechanism distinct from the NADH-dependent plasma membrane reductase. J. Biol. Chem. 1998; 273:13415–13420.
54. Halliwell B. Use of desferrioxamine as a 'probe' for iron-dependent formation of hydroxyl radicals. Evidence for a direct reaction between desferal and the superoxide radical. Biochem Pharmacol. 1985; 342:229–233.
55. Davis TA, Anderson EC, Ginsburg AV, Goldberg AP. Weight loss mproves lipoprotein lipid profiles in patients with hypercholesterolemia. J Lab Clin Med. 1985; 106:447-54
56. Inal MG, Kanbak G, Sunal. Antioxidant enzume activities and malondialdehyde levels related to ageing. Clin. Chim. Actam. 2001; 305:75-80
57. Sini N, Devi KS. Pharmaceutical biology. Antioxidant activities of the chloroform extract of Solanum trilobatum. 2004; 959:30-44.
58. Pastore A, Federici G, Bertini E, Piemonte F. Analysis of glutathione: implication in redox and detoxification. Clin Chim Acta. 2003; 333:19-39.
59. John S, Kale M, Rathore N, Bhatnagar D. Protective effect of vitamin E in dimethoate and malathion induced oxidative stress in rat erythrocytes. J Nutr Biochem. 2001; 12:500-504.
60. Sastre J, Asensi M, Gasco E, Pallardo FV, Ferrero JA, Furukawa T, Vina J. Exhaustive physical exercise causes oxidation of glutathione status in blood: prevention by antioxidant administration. Am J Physiol. 1992; 263:992-5.
61. Sah NK, Taneja TK, Pathak N, Begum R, Athar M and Hasnain SE. The baculovirus antiapoptotic p35 gene also functions via an oxidant-dependent pathway. Proc. Natl. Acad. Sci. USA. 1999; 96: 4838–4843.
62. Choe M, Jackson C, Yu BP, 1995. Lipid peroxidation contributes to age-related membrane rigidity. Free Radic Biol Med. 18:977-84.
63. Ishige K, Schubert D, Sagara Y. Flavonoids protect neuronal cells from oxidative stress
by three distinct mechanisms. Free Radic Biol Med. 2001; 30:433-46.
64. Myhrstad MC, Carlsen H, Nordstrom O, Blomhoff R, Moskaug JO. Flavonoids increase the intracellular glutathione level by transactivation of the gammaglutamylcysteine synthetase catalytical subunit promoter. Free Radic Biol Med. 2002; 32:386-93
65. Nakagawa T, Yokozawa T, Satoh A, Kim HY. Attenuation of renal ischemiareperfusion injury by proanthocyanidin-rich extract from grape seeds. J Nutr Sci Vitaminol (Tokyo). 2005; 51:283-6.
66. Saija A, Scalese M, Lanza M, Marzullo D, Bonina F, Castelli F. Flavonoids as antioxidant agents: importance of their interaction with biomembranes. Free Radic Biol Med. 1995; 19:481-6.
|