IJCRR - 9(3), February, 2017
Pages: 18-23
Date of Publication: 10-Feb-2017
Print Article
Download XML Download PDF
Prediction of the Protein 3D Structure of Fimbrial Protein Fim a Type 1 of Porphyromonas Gingivalis - Strain ATCC 33277(A Keystone Periodontal Pathogen) using Homology Modeling and Structure Analysis
Author: Priyanka K. Cholan, Judith Grace Jenefa
Category: Healthcare
Abstract:Introduction: Porphyromonasgingivalis, is a bacterium that has high degree of association with periodontitis, specially due to the expression of a wide array of virulence factors, of which the fimbriae is of particular interest owing to its adherence and colonization potential in the subgingival niche. This study deals with the determination of the protein 3D structure of major fimbriaefim A type-1of the pathogen Porphyromonasgingivalis\?strain ATCC 33277.
Materials Used: FASTA (Fast Adaptive Shrinkage Thresholding Algorithm) protein sequence from NCBI Database, Homology modeling server Swiss-model workspace, CASTp (Computed Atlas of Surface Topography of protein) server, Pro-Q (proetein quality predictor) and PROSESS ((Protein Structure Evaluation Suite and Server).
Methodology: The FASTA protein sequence of fimA type-1 of P. gingivalis\? strain ATCC 33277 was retrieved from NCBI database (NCBI- National Centre for Biotechnology Information). The 3D structures of the protein were determined by homology modeling server Swiss-Model workspace. Three models were predicted, and the most relevant structure is estimated by passing various quality assessments steps like ProQand validating test \?PROSESS
Results: The protein 3D modeling of major fimbriae fimA type-1 of the pathogen Porphyromonasgingivalis\?strain ATCC 33277were subjected to a series of quality check steps and the three most relevant models were prognosticated. In association with this, model 3 was considered to be the most valid and likely structure of fim A type 1.
Conclusion: This study paves way for future studies to be performed in this field including the identification of the protein structure and functions, its pathogenic role in periodontitis and thereby targeting the active sites and hence disease prevention.
Keywords: Fim A type-1, 3D structure, FASTA, Swiss model, CASTp, Prosess
Full Text:
INTRODUCTION
Porphyromonasgingivalis situs slot , a predominant black-pigmented anaerobic rod of the red complex group residing in subgingival biofilms, is widely recognized as a major contributor to the development of periodontal diseasesand other systemic infections, including coronary artery disease, stroke, diabetes mellitus, preterm delivery of low birth weight infants (1,2). P.gingivalis harbors many virulence factors including factors like fimbriae, haemagglutinin, capsule, lipopolysaccharide (LPS), outer membrane vesicles, organic metabolites such as butyric acid and various enzymes such Arg- and Lys-gigipains, collagenase, gelatinase, hyaluronidase and proteases(3). Among the various virulence factors -Fimbriae is a critical factor for colonization of P. gingivalis in the sub-gingival tissues and gingival crevices by the fimbriae-mediated adherence to the gingival epithelial cells (1).
Fimbriae are thin filamentous and proteinaceous surface appendages found in many bacterial species that plays an important pathogenic role in bacterial invasion. Fimbriae of P. gingivalis are composed of constituent (subunit) protein, fimbrillin, with a molecular weight of 40-42 kDa by sodium dodecyl sulfate-polyacrylamide gel electrophoresis.Ultra-structural examinations of these strains have shown that peritrichous fimbriae vary in length from 0.3 to 3 mm and are 5 nm wide (1,4) and have been classified as major or long fimbriae (FimA) based on their fimbrillin monomer configuration(5-7).
Lee et al.1991compared fimbriae diversities of size and amino terminal sequence of fimbrillins from various P. gingivalis strains; they differed in molecular weights rangingfrom 40.5 to 49 kDa and were classified into four types (types I to IV) based on the amino-terminal sequences of fimbrillins. Further molecular and epidemiological studies using PCRmethod to differentiate possibly varied bacterial pathogenicity revealed that P.gingivalis fimbriae are classified into six genotypes based on the diversity of the fim Agenes encoding each fimbrillin (types I to V, and type Ib (3,8).
The long fimbriae are primarily responsible for many of the adhesive properties of the organism, binding specifically to and activating various host cells, such as human epithelial, endothelial, and spleen cells, as well as peripheral blood monocytes, resulting in the release of inflammatory cytokines and several distinct adhesion molecules (9,10,11,12).
Currently, determining the three dimensional protein structures are of great significance in the field of medicine as many different types of biological experiments such as site-directed mutagenesis or structure-based discovery of specific inhibitors can be performed. Indeed, the number of structurally characterized proteins is small compared with the number of known protein sequences.
There are various methods of identifying the protein structures, including -a.genetic methods–site-directed mutagenesis, conceptual translation -b. Protein purification: chromatography, protein assay, gel electrophoresis, electro-focusing; -c. Advanced studies such as x-ray crystallography, protein NMR, cryo-electron microscopy, small angle scattering, etc. These methods seem to be extensive and very tedious. Thus computational methods for modeling 3D structures of protein have been developed to overcome these limitations.Some of them include: molecular dynamics, protein structural alignment, protein ontology. The number of possible folds in nature appears to be limited and the 3D structure of proteins are better conserved than their sequences, it is possible to identify a homologous protein with a known structure (template) for a given protein sequence (target). In these cases, homology modeling has proven to be the preferred method of choice to generate a dependable 3D model of a protein from its amino acid sequence as impressively shown in several meetings of the bi-annual situs judi slot online deposit pulsa tanpa potongan experiment. Hence this study was aimed at the identification of the protein3D structure of the major fimbriae fimA type-1 of Porphyromonasgingivalis strain ATCC 33277, by using homology modeling.
MATERIALS AND METHODOLOGY
(i) Homology modeling
Homology modeling is routinely used in many applications, such as virtual screening, or rationalizing the effects of sequence variations. To build a homology model, one must follow the following four fundamental steps:
(1) Identifying of the structural template(s),
(2) Aligning the target sequence and template structure(s),
(3) Building a model and
(4) Evaluating the quality of the model.
These steps can be repeated until a satisfying modeling result is achieved. Each of the four steps requires specialized software as well as access to up-to-date protein sequences and structure databases (13).
Further research in homology modeling brings out the use of seven detailed steps including,
1. Template recognition and initial alignment
2. Alignment correction
3. Backbone generation
4. Loop modeling
5. Side-chain modeling
6. Model optimization
7. Model validation (14)
(ii) FASTA- Sequence Alignment Program
For performing the first step in homology modeling, simple sequence alignment programs are used. In this case, modeling of P. gingivalis - fim-A protein is performed by the Fast Adaptive Shrinkage Thresholding Algorithm, FASTA which was first developed by Pearson and Lipman. This compares the test sequence and the query sequence and helps in formatting a template, which in turn provides us with a sequence.
(iii) SWISS-MODEL Workspace
With this sequence, further modeling is done using the SWISS-MODEL.There are various modeling modes in the SWISS-MODEL and the mode “My workspace” is used here. SWISS-MODEL workspace is an integrated Web-based modeling expert system. For a given target protein, a library of experimental protein structures is searched to identify relevant templates. On the basis of a sequence alignment between the target protein and the template structure, a three-dimensional model for the target protein is procured.The template structure database used by this workspace is derived from the Protein Data Bank (15). Thus homology modeling with SWISS-MODEL workspace has proved to be effective in determining the 3D protein structure of fim A of P. gingivalis and the following models were obtained- (refer figure - 1)
(iv)CASTp- Computed Atlas of Surface Topography of proteins
The active sites or pockets in the protein were identified by using CASTp and are highlighted with green in the models. (Refer figure -2). The proteins function through certain sites and hence recognition and identification of these active sites is essential to understand the function of the proteins. These active sites can be inhibited, which will in-turn reduce the action of the bacteria.
(v) ProQ – protein quality predictor
ProQ is a software to check the quality of the obtained model (refer figure 3). If the predicted structure satisfies the validation parameters of ProQ then the structure was taken for further analysis. The following results were obtained for the various models. (refer figure -3)
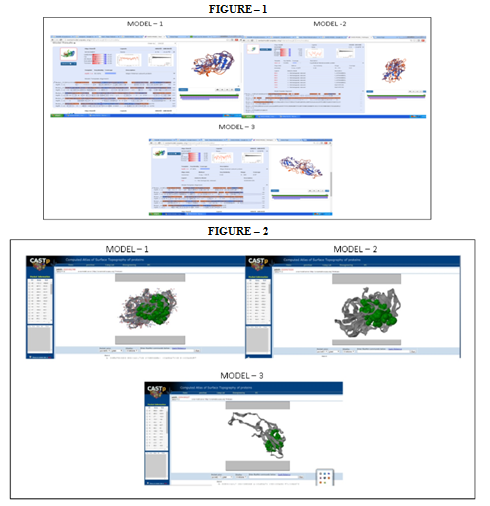
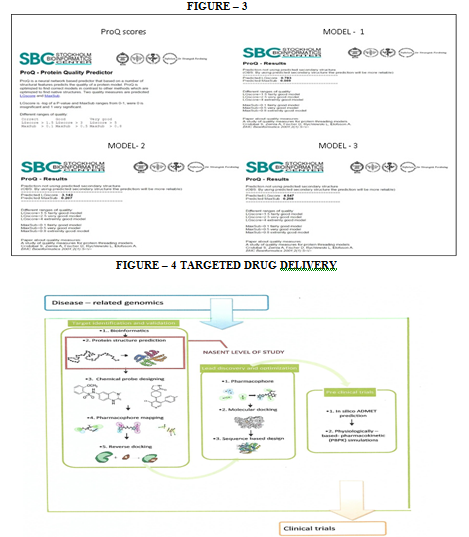
(vi) PROSESS- Protein Structure Evaluation Suite and Server
Model-3 is the one,which shows much higher scores when compared to the other models. Thus this model was further assessed by Protein Structure Evaluation Suite and Server, PROSESS, to validate the model obtained.
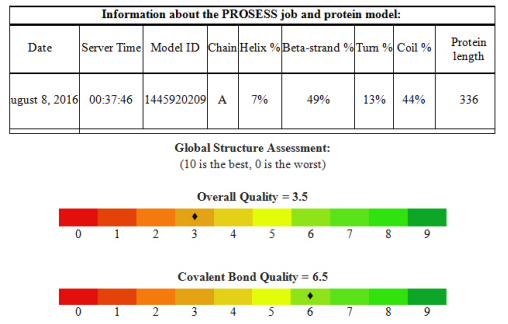
The covalent bonds/peptide bonds play an important role in determining the shape of the protein that is very important for its function. The bond links the adjacent amino acid residues inaproteinformed by condensation reaction between the amine group of one amino acid and the carboxyl group of another with the release of a water molecule. These bonds are highly specific, thus they are important in determining the structure of the protein. (16)

The non-covalent bonds also referred to as interactions are weak bonds and maintains the 3D structure of the large protein molecules. Their existence is transientand multiple bonds act together to produce highly stable and specific associations between different parts of a large molecule. (17)

These angles are important local structural parameters that control protein folding and provide the flexibility required for the polypeptide backbone to adopt a certain fold. Thus they provide insights into the function of the protein.
DISCUSSION
The function of the protein depends on its structure. The structure in-turn depends on the physical and chemical parameters. Although the information needed for life is encoded by the DNA molecule, the dynamic process of life in maintenance, replication, defense and reproduction are carried out by these proteins. Thus obtaining the three dimensionalproteinstructure provides us with information about the medicinally relevant receptors, small ligands, etc and targeting this protein in the pathogen for therapy is essential with many benefits, including, decreasing the dosage of the drug, reduced adverse effects, low rates of drug toxicity, faster rates of action and definitive outcomes. Drugs are ligands that not only fit onto the binding pocket of the target protein, but are also absorbed, transported, distributed to the right compartment. It is highly stable to metabolization, safe, free of side-effects and chemically stabile in the formulation. Finding a lead compound, optimizing its properties and obtaining a drug takes enormous time and money. This in turn is simplified by varied molecular modeling tools (18) (refer figure 4). The current study which is at its nascent level dealing with targeted drug delivery has promising results down the road.
Any protein structure has four levels including primary, secondary, tertiary and quaternary structures respectively. Primary structure is the linear sequence of amino acids. Secondary structure is the local con-formation of α-helixes, β-sheets and random coils. The angle between two adjacent amino acids is called torsion angle, which deter-mines the twists/turns of the sequences resulting in secondary structure. Tertiary structure (3D) results from the packing of secondary structural elements at a stable conformation. Quaternary structure is combination of one or more subunits or chains. (18). Obtaining an accurate model through the conventional techniques such as NMR (nuclear magnetic resonance spectroscopy) analysis or X-ray diffraction techniques is time consuming and elaborate. Thus homology modeling proves to be one of the most valid and efficient means of obtaining an accurate model of protein.
And so, inthis study, the protein 3D structure of fimA type 1 of P. gingivalis was found by using the afore mentioned technique of SWISS MODEL workspace. This included obtaining the FASTA sequence by using the amino-acid sequence, which was then used for homology modeling from which the three models were obtained (refer figure 1). Model quality assessment tools are used to evaluate the reliability of the resulting models. With the help of CASTp, the active sites were predicted for the three protein models (refer figure 2). These structures obtained were validated by ProQ and the LG scores were 0.783, 3.142 and 4.547 respectively and the Max Sub scores were 0.059, 0.207 and 0.298 respectively (refer figure 3).Subsequently, model 3 was considered the finest model and with this model further analysis was made. PROSESS was done to assess the quality of the structure obtained and the results were tabulated in the above mentioned manner (refer results). The values of the covalent bond quality showed a high score of 6.5. But certain values related to the non-covalent bond quality and the torsion angle qualityhowever, showedlower values of about 3.5. This becomes a limitation of the protein modeling of fim A type 1 of P.gingivalis and further laborious studies are required to validate the above results and predict the appropriate structure making use of our analysis.
This homology modeling technique is currently the most meticulous and time saving computational method to generate reliable structural models and is frequently used in many biological scenarios. Normally, the computational effort for a modeling project is fairly less and lasts only for a few hours. However, this does not include the time required for visualization and interpretation of the model, which may vary depending on the personal experience working with protein structures.
Thus this study has resulted in the identification of the three dimensional protein model of fimA type 1 of P.gingivalis –strain ATCC 33277, which is an extremely good model – a model verified and validated by many tests. This study is only the beginning of an elaborate and extensive research work that must be carried out to aid in the discovery of targeted drugs and other substancesthat can inactivate the periopathogen at the very germinal stage of adhesion to the host.
CONCLUSION
Thus, from the above modeling performed andafter being subjected through a series of analysis, MODEL-3 was found to be more accurate when compared to the other models obtained. Thus avalidated three dimensional model or a protein structure of the fim-A type-1 of P.gingivalis isobtained through homology modeling. Identifying this protein structure is only the initial step for manifoldrigorous time-consuming procedures including identifying and proving the functions of the protein and its pathogenic role in periodontitis and finally targeted drug delivery. Even though targeted drug delivery seems to be one of the leading ways in therapy for a myriad of diseases, its role in periodontitis is still questionable and continues to remain a myth whatsoever!!
ACKNOWLEDGEMENTS
Authors acknowledge the immense help received from the scholars whose articles are cited and included in references of this manuscript. The authors are also grateful to authors / editors / publishers of all those articles, journals and books from where the literature for this article has been reviewed and discussed.
Conflicts of interest: None
Source of Funding: None
References:
1. Morten Enersen1, Kazuhiko Nakano, Atsuo Amano. Porphyromonasgingivalis fimbriae . J Oral Microbiol 2013; 5: 20265.
2. Holt S, Ebersole J. Porphyromonasgingivalis, Treponemadenticola, and Tannerella forsythia: the ‘‘red complex’’, a prototype polybacterial pathogenic consortium in periodontitis. Periodontol 2000 2005; 38: 72-122.
3. Shigenobu Kimura, Yuko Ohara-Nemoto, Yu Shimoyama, Taichi Ishikawa and Minoru Sasaki.Pathogenic Factors of P. gingivalisand the Host Defense Mechanisms.Intechopen 2012; 1 : 1-17.
4. Lamont RJ, Jenkinson H. Life below the gum line: pathogenic mechanisms of Porphyromonasgingivalis. MicrobiolMolBiol Rev 1998; 62: 1244-63.
5. Dickinson DP, Kubiniec MA, Yoshimura F, GencoRJ.Molecular cloning and sequencing of the gene encoding the fimbrial subunit protein of Bacteroidesgingivalis. J Bacteriol 1988; 170: 1658-65.
6. Lee JY, Sojar HT, Bedi GS, Genco RJ. Porphyromonas (Bacteroides) gingivalisfimbrillin: size, amino-terminalsequence, and antigenic heterogeneity. Infect Immun 1991; 59: 383-9.
7. Wu H, Fives-Taylor P. Molecular strategies for fimbrial expression and assembly. Crit Rev Oral Biol Med 2001; 12: 101-15.
8. Slots J, Gibbons RJ. Attachment of Bacteriodes melaninogenicus subsp. asaccharolyticus to oral surfaces and its possible role in colonization of the mouth and periodontal pockets. Infect Immun 1978; 19: 254-64.
9. Holt S, Ebersole J. Porphyromonasgingivalis, Treponemadenticola, and Tannerella forsythia: the ‘‘red Complex’’, a prototype polybacterial pathogenic consortium in periodontitis. Periodontol 2000 2005; 38: 72-122.
10. Lamont RJ, Jenkinson HF. Subgingival colonization by Porphyromonasgingivalis. Oral Microbiol Immunol 2000; 15: 341-9.
11. Amano A, Nakagawa I, Okahashi N, Hamada N. Variations of Porphyromonasgingivalis fimbriae in relation to microbial pathogenesis. J Periodontal Res 2004; 39: 136-42.
12. Amano A. Molecular interaction of Porphyromonasgingivalis with host cells: implication for the microbial pathogenesis of periodontal disease. J Periodontol 2003; 74: 90-6.
13. Konstantin Arnold, Lorenza Bordoli, Ju¨ rgenKopp and TorstenSchwede. The SWISS-MODEL workspace: a web-based environmentfor protein structure homology modeling. Bioinformatics 2006; 22: 195–201
14. Elmar Krieger, Sander B. Nabuurs, and GertVriend. Homology modeling. 2003; pages 507-521.
15. Lorenza Bordoli, Florian Kiefer, Konstantin Arnold, Pascal Benkert, James Battey and TorstenSchwede. Protein structure homology modeling using SWISS-MODEL workspace. Nature Protocols 2008; 4: 1 – 13.
16. Ardala Breda, Napoleao Fonseca Valadares, Osmar Norberto de Souza, and Richard Charles Garratt. Protein structure, Modelling and Applications. Chapter A06, September 14, 2007; Pages1-185.
17. S. Brindha, Sangzuala Sailo, Liansangmawii Chhakchhuak, Pranjal Kalita, G. Gurusubramanian , N. Senthil Kumar.Protein 3D structure determination using homology modeling and structure analysisSci Vis 2011; 125-133.
18.Lodish H, Berk A, Zipursky SL, et al. Molecular Cell Biology- Purifying, Detecting and Characterizing. 5th edition; Section 3.6: Pages 86-99.
19.Socransky SS, Haffajee AD, Cugini MA, Smith C, KentRL. Jr. Microbial complexes in subgingivalplaque. J. Clin Periodontol 1998; 25: 134-44.
20. Socransky SS, Haffajee AD. Periodontal microbial ecology. Periodontol 2000 2005; 38: 135-87.
21. Dzink JL, Socransky SS, Haffajee AD. The predominant cultivable microbiota of active and inactive lesions of destructiveperiodontal diseases. J Clin Periodontol 1988; 15: 316-23.
22. Frandsen EV, Poulsen K, Curtis MA, Kilian M. Evidence of recombination in Porphyromonasgingivalis and random distributionof putative virulence markers. Infect Immun 2001; 9:4479-85.
23. Okuda K, Slots J, Genco RJ. Bacteroidesgingivalis, Bacteroidesasaccharolyticus and Bacteroidesmelaninogenicus subspecies: cell surface morphology and adherence to erythrocytes andhumanbuccal epithelial Cells. Curr Microbiol 1981; 6: 7-12.
24. Handley PS, Tipler LS. An electron microscopic survey of thesurface structures and hydrophobicity of oral and non-oral species of the bacterial genus Bacteroides. Arch Oral Biol 1986;31: 325-35.
|