IJCRR - 8(9), May, 2016
Pages: 01-06
Date of Publication: 12-May-2016
Print Article
Download XML Download PDF
OXIDATIVE STRESS BIOMARKERS IN RATS EXPOSED TO BROMOXYNIL
Author: Ahmed K. Salama, Khaled A. Osman, Ahmed S. El-Bakary, Maher S. Salama
Category: Healthcare
Abstract:Aim: The present study was designated to evaluate the oxidative stress biomarkers in male rat following oral repetitive administration of 0.1 LD50 of the herbicide bromoxynil. Methodology: Animals were orally received four doses of 0.1 LD50 of bromoxynil every other day. Twenty-four hours after the last oral dosing, all rats were killed by decapitation. Blood, brain, liver, and kidneys were taken for determination of TBARS, lactic dehydrogenase, catalase and alkaline phosphatase. Results: TBARS were found to be significantly increased in the liver, kidneys and brain where they were 390.20, 293.80, and 287.03% of control, respectively. In case of serum it was insignificantly increased to 162.88% of control value. Lactic dehydrogenase activity was significantly enhanced in serum and liver comparing with the control values (119.49 and 114.12%) and insignificantly enhanced in kidneys and brain (105.17 and 107.40%). Catalase activity was increased in all tissues where the enhancement was significant in both of serum and liver (122.68 and 119.99%, respectively) and insignificant in case of kidneys and brain (112.55 and 105.12%, respectively). Alkaline phosphatase activity in serum, liver, kidneys and brain was found to be elevated. These values were significant in liver and kidneys (113.47 and 121.14%, respectively) while they were insignificant in serum and brain (109.91 and 114.46%, respectively). Conclusion: Therefore, the herbicide bromoxynil could produce significant alteration in the lipid peroxidation and activities of some antioxidant enzymes and producing cellular oxidative damage in male rats following repetitive oral dosing.
Keywords: Bromoxynil, Oxidative stress, Rat, Biomarkers, Antioxidant, Enzymes
Full Text:
INTRODUCTION Pesticides are used to prevent, control and eliminate unwanted pests and associated diseases. However, large amounts of these compounds caused environmental and public health concerns (Bhanti et al, 2007). Pesticide intoxication induces a derangement of certain antioxidant mechanisms in different tissues, including alterations in antioxidant enzymes and the glutathione redox system (Ahmed et al, 2001). Exposure to pesticides can induce oxidative stress by the induction of reactive oxygen species (ROS) as byproducts of detoxifying metabolism, by alteration in antioxidant defense mechanisms, including detoxification and scavenging enzymes, or by increasing lipid peroxidation as a result of the interaction between reactive oxygen species (ROS) and cellular or subcellular membranes (Abdollahi et al., 2004).
These mediate a wide variety of toxic effects such as DNA damage or genotoxicity (Ryter et al, 2007 and Franco et al, 2009). Overproduction of reactive oxygen and nitrogen species can result from exposure to environmental pollutants (Poljsak and Fink, 2014). Oxidative stress arises if detoxification systems and antioxidants are compromised or if ROS production is excessive, resulting in DNA, protein and lipid oxidation (Ryter et al, 2007, West and Marnett, 2006, Franco et al, 2007). A more recent pilot study has pointed out that oxidative stress and DNA damage are possibly linked to pesticides-induced adverse health effects in agricultural workers (Muniz, 2008). Bromoxynil (3,5-dibromo-4-hydroxybenzonitrile) is used for the post-emergence control of annual broad-leaved weeds in cereals, maize, sorghum, flax, allium species, mint, and grass seed crops (Roberts, 1998).
Bromoxynil is active as mitochondrial uncoupler (Tomlin, 2000). Bromoxynil is highly toxic to mammals and birds. The data for the acute oral LD50 in rats range from 190 mg/kg up to 440 mg/kg (Krieger et al, 2001). Recently, data indicate that the toxic action of pesticides may include the induction of oxidative stress and accumulation of free radicals in the cell. A major form of cellular oxidation damage is lipid peroxidation, which is initiated by hydroxyl free radical through the extraction of hydrogen atom from unsaturated fatty acids of membrane phospholipids (Farber et al., 1990). As a consequence, these compounds can disturb the biochemical and physiological functions of cells in blood and liver (Akhgari et al., 2003).
The increased oxidative stress resulted in an increase in the activity of antioxidant enzymes such as superoxide dismutase and catalase. The enhancement of release of lactate dehydrogenase (LDH) is also indicative of cellular and membrane damage, while inactivation of superoxide dismutase and catalase are expected to enhance the generation of reactive oxygen species (ROS) and consequently, pose an oxidative stress upon the system (Tabatabaie and Floyed, 1996). The production of ROS can initiate lipid peroxidation and cause intracellular excess of malondialdehyde (MDA) (Peluso et al, 2010). Astiz et al (2011) found that thiobarbituric acid-reactive substances were increased in the exposed workers group of professional sprayers to various agrochemicals for about 10 years.
Our previous studies investigated the ability of many different groups of pesticides to induce oxidative stress in animals (Osman, 1999, Osman et al, 2000, Salama et al, 2005 and Salama et al 2013). The aim of the present study was to investigate the effect of the herbicide bromoxynil on producing alteration in the lipid peroxidation and activities of some antioxidant enzymes and producing cellular oxidative damage in male rats following repetitive oral dosing.
MATERIALS AND METHODS Chemical Bromoxynil (3,5-dibromo-4-hydroxybenzonitrile) was supplied by Chem Service, West Chester (99% purity). All other chemicals used in this study were obtained either from Sigma or BDH Companies and they were of the highest grade available. Animals Male rats, Ratus norvegicus, weighing an average of 110 g were obtained from the High Institute of Public Health, Alexandria University. Animals were randomly separated and housed in stainless steel cages (5 per cage) and left two weeks under the laboratory conditions. Animals were provided rodent chow and tap water ad libitum through the study. Treatment of animals Male rats were divided into two groups, five rats each. The first group was orally received four doses of 0.1 LD50 of bromoxynil every other day.
The second group received corn oil as the same manner of pesticide treated animals and served as vehicle control. Twenty-four hours after the last oral dosing, all rats were killed by decapitation. Blood samples were taken using non-heparinized tubes. Blood was centrifuged at 5000 rpm for 5 min at 4 °C to separate serum for analysis of oxidative stress biomarkers. Brain, liver, and kidneys were excised rapidly, weighed, placed in glass vials and stored at -20 °C until determination. Preparation of tissue homogenate Selected tissues were homogenized in saline solution (1:10 w/v) using a polytron homogenizer. The homogenates were used for determination of thiobarbituric acid reactive substances (TBARS), lactic dehydrogenase, catalase and alkaline phosphatase. Lipid Peroxidation assay The determination of lipid peroxidation was based on the formation of thiobarbituric acid-reactive substances (TBARS) (Uchiyama and Mihara, 1978).
To 0.5 ml of the homogenate, 3 ml of 1% phosphoric acid and 1 ml of 0.6% thiobarbituric acid aqueous solution were added. The mixture was heated for 45 min in a boiling water bath and then cooled. n-Butanol (4 ml) was added to the above mixture and mixed vigorously. The butanol layer was measured was separated by centrifugation and the absorbance was measured at 532 and 520 nm. Malondialdehyde (MDA) was employed as the standard and the molar absorptivity constant of 1.56 x 10-5 M cm-1 was used. Lipid peroxidation is expressed as nmole MDA/mg protein. Lactic dehydrogenase assay The determination of lactic dehydrogenase (LDH) was based on the conversion of lactate to pyruvate or pyruvate to lactate (Moss et al, 1986). The rate of NADH oxidation is proportional to LDH activity. Twenty microliter of tissue homogenate was added to 1ml of 50 mM phosphate buffer pH 7.5 containing o.6 mM sodium pyruvate, 0.9 g/L sodium azide and 0.18 mM NADH. The mixture was gently mixed and incubated at 30 °C. The rate of NADH oxidation was measured at 340 nm. LDH activity is expressed as unit.mg protein-1.
Catalase assay Catalase activity was assayed by the method of Aebi (1984). The decrease in absorbance was measured at 240 nm. The enzyme activity was expressed as unit of catalase .mg-1 protein. One unit of catalase was defined as the amount of enzyme necessary to reduce 1 µmole of H2 O2 per minute. Alkaline phosphatase assay Alkaline phosphatase activity was estimated according to McComb et al (1966) using sodium p-nitro phenyl phosphate as a substrate.
Determination of protein The protein contents were determined by the method of Lowry et al (1951), using bovine serum albumin as standard. Statistical analysis Data were calculated as mean ±S.D. and analyzed using analysis of variance technique (ANOVA) followed by Least Significant Difference (LSD). Probability of 0.05 or less was considered significant. All statistical analysis was done with Costat Program (1986) on a personal computer.
RESULTS Effect of bromoxynil on lipid peroxidation Lipid peroxidation levels were determined in different tissues of male rats following oral administration with repetitive doses of 12.9 mg/kg b.wt. (0.1 LD50) of bromoxynil. The concentrations of thiobarbituric acid-reactive substances (TBARS) in male rats intoxicated with bromoxynil are presented in Table (1). TBARS concentrations were significantly increases in the liver, kidneys and brain where they were 390.20, 293.80, and 287.03% of control, respectively. While in case of serum it was insignificantly increased to 162.88% of control value. Effect of bromoxynil on the activity of lactic dehydrogenase (LDH) Lactic dehydrogenase activity in serum, liver, kidneys and brain were measured following bromoxynil administration and presented in Table (2).
The results clearly indicate that bromoxynil induced oxidative stress, where serum and liver LDH activities were significantly increased comparing with the control values. They were 119.49 and 114.12% of control in serum and liver, respectively. Lactic dehydrogenase activity also insignificantly increased in kidneys and brain. The values were 105.17 and 107.40% of control in kidneys and brain, respectively. Activity of catalase The increased oxidative stress resulted in an increase in the activity of antioxidant enzymes such as catalase is indicative of cellular and membrane damage. Catalase activity in different tissues of male rat was assayed following oral administration with repetitive doses of 12.9 mg/kg of bromoxynil (Table 3).
Our findings indicated that the catalase activity was increased in all tissues where the enhancement was significant in both of serum and liver (122.68 and 119.99%, respectively) and insignificant in case of kidneys and brain (112.55 and 105.12%, respectively). Activity of alkaline phosphatase The repetitive oral administration of 12.9 mg/kg of bromoxynil to male rats caused an enhancement of alkaline phosphatase activity in serum, liver, kidneys and brain. These values were significant in liver and kidneys (113.47 and 121.14%, respectively) while they were insignificant in serum and brain (109.91 and 114.46%, respectively) (Table, 4).
DISCUSSION The obtained results indicate that the repetitive exposure to the herbicide, bromoxynil lead to the generation of oxygenated reactive species (ROS) which affects the activity of the scavenging enzyme system. Overproduction of reactive oxygen and nitrogen species can result from exposure to environmental pollutants, including pesticides (Poljsak and Fink, 2014). The production of ROS can initiate lipid peroxidation and cause intracellular excess of malondialdehyde (MDA) (Peluso et al, 2010). The potential of ROS to damage tissues and cellular components is known as oxidative stress.
Astiz et al (2011) found that thiobarbituric acidreactive substances were increased in the exposed workers group of professional sprayers to various agrochemicals for about 10 years. In our study, lipid peroxidation, lactic dehydrogenase, catalase and alkaline phosphatase were assayed and considered as good biomarkers for oxidative stress. The results indicate that the toxic action of pesticides include the induction of oxidative stress and accumulation of free radicals in the cell. A major form of cellular oxidation damage is lipid peroxidation, which is initiated by hydroxyl free radical through the extraction of hydrogen atom from unsaturated fatty acids of membrane phospholipids (Farber et al., 1990). As a consequence, these compounds can disturb the biochemical and physiological functions of cells in blood and liver (Akhgari et al., 2003). Our results concerning the lipid peroxidation levels indicate that the level of MDA increment is pesticide dependent. Evidence from in vivo studies with many toxicants including pesticides supports the concept that free radicals e.g. hydroxyl radicals .
OH, H2 O2 and others, are important mediators of tissue injury and formation of these radicals result in increased lipid peroxidation (Farber et al., 1990; Akhgari et al., 2003, Jaiswal et al, 2014). Shvedova et al (2003) studied the oxidative stress, inflammatory biomarkers, and toxicity in mouse lung and liver after Inhalation exposure to diesel emissions. They showed that the degree of cytotoxicity/tissue damage and inflammation was evaluated by assessing the LDH and they observed a significant increase in the levels of LDH compared to control. The increased oxidative stress resulted in an increase in the activity of antioxidant enzymes such as catalase.
The enhancement of release of lactate dehydrogenase (LDH) is also indicative of cellular and membrane damage (Tabatabaie and Floyed, 1996, Osman, 1999, Osman et al, 2000, Salama et al, 2005 and Salama et al 2013). Catalase detoxifies H2 O2 in the biological systems. Hermes-Lima (2004) indicated that catalase activity often increase due to the regulation by ROS. Alkaline phosphatase is involved in trans phosphorylation reactions. The increase in this enzyme activity is attributed to its release from ruptured cells due to the effect of pesticides exposure (Shaffi, 1980).
CONCLUSION The current study indicates that the repetitive exposure to bromoxynil herbicide could produce significant alteration in lipid peroxidation and some antioxidant enzyme activities such as lactic dehydrogenase, catalase and alkaline phosphatase. The results demonstrated that intoxication with Bromoxynil induced significant damage of the serum, brain, liver and kidneys tissues leading to imbalance in enzymes activities in different organs and could produce oxidative damage.
ACKNOWLEDGEMENT Authors acknowledge the immense help received from the scholars whose articles are cited and included in references of this manuscript. The authors are also grateful to authors / editors / publishers of all those articles, journals and books from where the literature for this article has been reviewed and discussed.
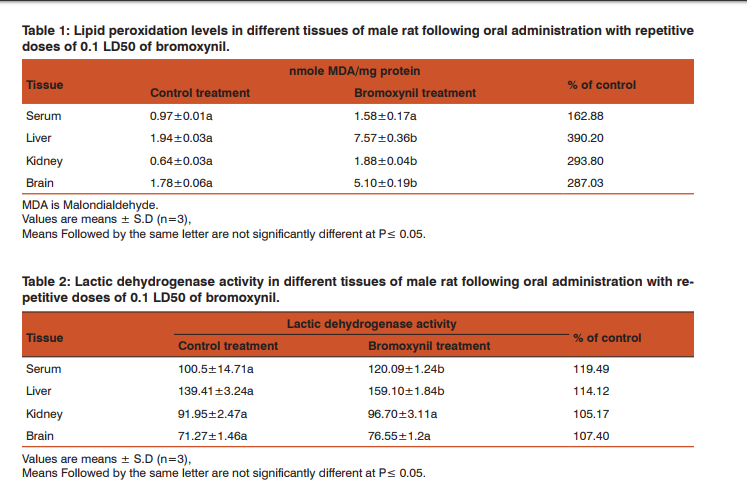
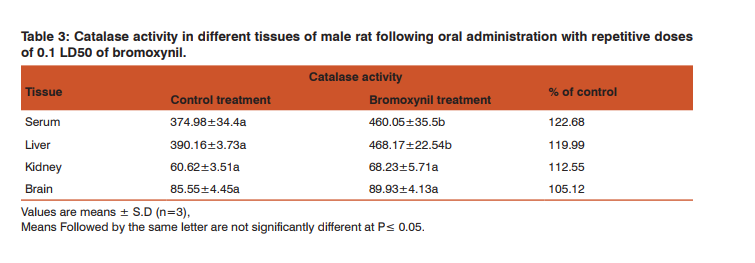
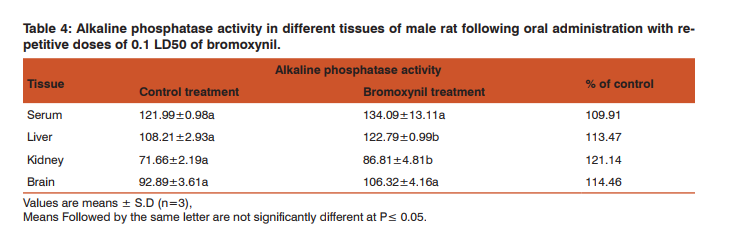
References:
1. Abdollahi, M., Ranjbar, A., Shadnia, S., Nikfar, S.; Rezaiee, A., 2004. Pesticides and oxidative stress: a review. Med. Sci. Monit., 10: 141–147.
2. Aebi, H., 1984, Catalase in vivo. Methods Enzymol. 105, 121- 126.
3. Akhgari, M. , Abdollahi, M. , Kebryaeezadeh, A., Hosseini, R. Sabzevari , O. 2003, Biochemical evidence for free radical-induced lipid peroxidation as a mechanism for subchronic toxicity of malathion in blood and liver of rats. Hum Exp Toxicol., 22(4):205-11.
4. Astiz, M., Arnal, N., de Alaniz, M.J. and Marra, C.A. , 2011, Occupational exposure characterization in professional sprayers: Clinical utility of oxidative stress biomarkers. Environ. Toxicol. Pharmacol., 32(2):249-258.
5. Banerjee, B.D., Ahmed, R.S., 2001. Pesticide-induced oxidative stress: perspectives and trends. Rev Environ Health.16(1):1-40.
6. Bhanti, M., Taneja, A., 2007, Contamination of vegetables of different seasons with organophosphorus pesticides and related risk assessment in northern India. Chemosphere, 69(1):63-68.
7. Costat Program, 1986, Version 2, Cohort Software.
8. Farber, J.L., Kyle, M.E. , Coleman, J.B., 1990, Biology of disease: Mechanisms of cell injury by activated oxygen species. La. Inves. 62:670-679.
9. Franco, R., Sanchez-Olea, R., Reyes-Reyes, E.M., Panayiotidis, M.I., 2009, Environmental toxicity, oxidative stress and apoptosis: ménage a trois. Mutat. Res., 674:3-22.
10. Franco, R., Schoneveld, O.J., Pappa, A., Panayiotidis, M.I., 2007, The central role of glutathione in the pathophysiology of human diseases. Arch. Physiol. Biochem., 113:234-258.
11. George, N., Bowers, Jr., McComb, R.B., 1966. A Continuous Spectrophotometric Method for Measuring the Activity of Serum Alkaline Phosphatase. Clinical Chemistry, 12(2): 70-89.
12. Hermes-Lima, M., 2004, Oxygen in biology and biochemistry: role of free radicals. In: Storey, K.B. (Ed.), Functional metabolism: Regulation and adaptation. John Wiley and Sons, New York, pp. 319-368.
13. Jaiswal, S.K., Siddiqi, N.J., Sharma, B., 2014, Carbofuran induced oxidative stress mediated alterations in Na+ -K+ -ATPase activity in rat brain: amelioration by vitamin E. J. Biochem. Mol. Toxicol., 28(7):320-7.
14. Krieger, R., Doull, J., Ecobichon, D., Gammon, D., Hodgson, E., Reiter, L. Ross, J. 2001, Handbook of Academic Toxicology Principles, Academic Press.
15. Lowry O.H., Rosebrugh, N.J., Farr , A.L. , Randall, R.J. , 1951, Protein measurement with the Folin phenol reagent. J. Biol. Chem. 193:265- 275.
16. Moss, D.W., Henderson, A.R. , Kachmar, J.R. , 1986, Enzymes. In: Textbook of Clinical Chemistry (N.W. Tietz, Ed.), W.B. Saunders, Philadelphia, PA. pp:691-663.
17. Muniz, J.F., McCauley, L., Scherer, J., Lasarev, M., Koshy, M., Kow, Y.W., Nazar-Stewart, V., Kisby, G.E., 2008, Biomarkers of oxidative stress and DNA damage in agricultural workers: a pilot study. Toxicol. Appl. Pharmacol., 227(1):97-107
18. Osman, K.A., Aly, N.M. , Salama, A. K. , 2000, The role of vitamin E and glutathione as antioxidants in the protection of oxidative stress induced by paraquat and diquat in female rats. Alex. Sci. Exch., vol 21 : 247-259.
19. Osman, K.A.,1999, Lindane, chlorpyrifos and paraquat induced oxidative stress in female rats. Alex.J. Res., 44:345-355.
20. Peluso, M., Srivatanakul, P., Munnia, A., Jedpiyawongse, A., Ceppi, M., Sangrajrang, S., Piro, S., Boffetta, P. 2010, Malodialdehyde-Deoxyguanosine adducts among workers of Thai industerial estate and nearby residents. Environ. Health Perspect. 118(1), 55-59.
21. Poljsak, B., Fink, R. , 2014, The protective role of antioxidants in the defence against ROS/RNS-mediated environmental pollution. Oxid Med Cell Longev., 2014:671539.
22. Roberts, T. R., Huston, D. H., Lee, P. W., Nicholls, P. H., Plimmer, J. R., 1998, Metabolic pathways of agrochemicals. Part 1, Herbicides and plant growth regulators. The Royal Society of Chemistry, Cambridge, UK.
23. Ryter, S.W., Kim, H.P., Hoetzel, A., Park, J.W., Nakahira, K., Wang, X., Choi, A.M., 2007, Mechanisms of cell death in oxidative stress. Antioxid. Redox Signal. 9:49-89
24. Salama A.K., Osman, K. A., Ahmed, N. S. , Soliman, S.A. ,2005, Oxidative stress induced by different pesticides in the land snails Helix aspersa. Pak. J. Biol. Sci 8 (1), 92-96.
25. Salama, A.K., Osman, K. A., Omran, O.A. , 2013, Pesticidesinduced oxidative damage: Possible in vitro protection by antioxidants. Journal of Toxicology and Environmental Health Sciences, 5(5):79-85.
26. Shaffi, S.A., 1980, Thiodon toxicity: non-specific phosphomonoesterases in nine freshwater teleosts. Toxicol. Letters, 6:339- 347.
27. Shvedova, A.A., Yanamala, N., Murray, A.R., Kisin, E.R., Khaliullin, T., Hatfield, M.K., Tkach, A.V., Krantz, Q.T.,Nash, D., King, C., Gilmour , M.I., Gavett, S.H. 2013, Oxidative Stress, Inflammatory Biomarkers, and Toxicity in Mouse Lung and Liver after Inhalation Exposure to 100% Biodiesel or Petroleum Diesel Emissions. J. Toxicology and Environmental Health A, 76 (15): 907-921.
28. Tabatabaie T, Floyed, R.A., 1996, Inactivation of glutathione peroxidase by benzaldehyde. Toxicol. Appl. Pharmacol. 141: 389-393.
29. Tomlin, C.D. (2001): “The pesticide manual” 12th ed, British Crop Protection.
30. Uchiyama, M. and Mihara,M., 1978, Determination of malondialdehyde precuesor in tissue by thiobarbaturic acid test. Anal. Biochem., 86:271-278.
31. West, J.D., Marnett, L.J., 2006, Endogenous reactive intermediates as modulators of cell signaling and cell death. Chem. Res. Toxicol., 19:173-194.
|