IJCRR - 3(9), September, 2011
Pages: 11-20
Print Article
Download XML Download PDF
A REVIEW ON IONIC LIQUIDS
Author: Tryambake M.U., Kuchekar B. S., Chabukswar A.R., Lokhande P. D., Kadam V.J., Tryambake M .B
Category: General Sciences
Abstract:Ionic liquids are the salts that are liquid at the low temperature and their molten forms composed of the ions. Their usefulness can be due to favorable physicochemical properties, like the lack of vapors pressure, good thermal and chemical stability as well as very good dissolution properties regarding both organic and inorganic compounds. The review begins with a discussion of the earliest successful study of ionic liquids (ILs) for their nomenclature, preparations; effect of properties on ionic liquids, uses and potential applications in organic and analytical chemistry continues to grow.
Keywords: Ionic Liquids (ILs), inorganic salt, electrochemical stability, asymmetric amide
Full Text:
1. INTRODUCTION
Particularly the ionic liquids are used as the media in various reactions including cracking, dissolution, hydrogenation, dimerization, isomerization, oligomerisation,and other reactions. They are used in catalyst systems or reactions to show better activity, selectivity and stability. They provide better yield and in some cases faster kinetics. They particularly have properties like Hydrophobicity, Viscosity, Electrochemical stability, Thermal stability. They provide greener reaction media hence provide environmentally friendly product.1 Ionic liquids have been described as designer solvents, and this means that their properties can be adjusted to suit the requirements of a particular process. Properties such as density2 , melting point3 , viscosity4 , Refractive index5 and Surface tension6 can be varied by simple changes to the structure of the ions. Nowadays ILs are applied in several areas of chemistry, like Wittig reaction7 Friedel crafts reaction8,9 , Diels- alder reaction10, Heak reaction11 , Suzuki coupling12 and (bio) catalytic reactions13 . The growing interest in molten salts is also visible in analytical chemistry, especially in LC and CE14 .
2. HISTORY
Ethanolammonium nitrate
(m.p. 52- 55°C) was reported in 1888 by S. Gabriel and J. Weiner. One of the earliest truly room temperature ionic liquids was ethyl ammonium nitrate (C2H5) NH3 + ·NO3 − (m.p. 12°C), synthesized in 1914 by Paul Walden. In the 1970s and 1980s there was interest in ionic liquids based on alkyl-substituted imidazolium and pyridinium cations, with halide or trihalogenoaluminate anions, initially developed for use as electrolytes in battery applications. In 1992, Wilkes and Zawarotko obtained ionic liquids with 'neutral' weakly coordinating anions such as hexafluorophosphate (PF6 − ) and tetrafluoroborate (BF4 − ), allowing a much wider range of applications for ionic liquids.
3. NOMENCLATURE OF THE IONIC LIQUIDS
On the basis of the anion, ILs may be divided into four groups: (a) systems based on AlCl3 and organic salts such as 1-butyl- 3-methylimidazolium chloride,[bmim][Cl]; (b) systems based on anions like [PF6] - ,[BF4] - and [SbF6] - , (c) systems based on anions such as[CF3SO3] - , [(CF3SO2)2N]- , [Tf2N]- and similar, (d) systems based on anions such as alkylsulfates1 , and alkylsulfonates15 . First group (a) called first generation. Lewis acidity can be varied by the relative amount of the organic salt/AlCl3 molar excess of the AlCl3 are Lewis acidic , excess of organic salt are Lewis basic. These are extreme hygroscopic and handling is possible on dry atmosphere. Second group (b) is nearly neutral and air stable, and they have advantage of reacting exothermically with strong Lewis acids. Third are characterized by low melting point, low viscosity, and high conductivities. [Tf2N] act as coordinating solvent, Structural studies of [Tf2N] and weak Lewis acids attribute to delocalization of the negative charge with S-N-S core 16. Alternative is to find low coordinating and non fluorinated anions such as [(CB11H12) - , (CB11H6Cl6) - , CB11H6Br6 - )] 17. Fourth are characterized by wide electrochemical window and air stability.
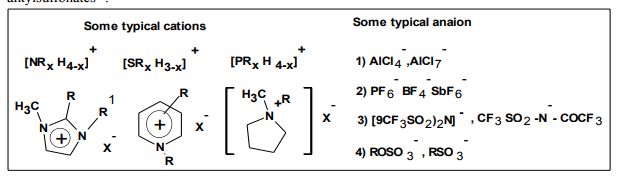
Cations: Generally cations of N, N dialkyl imidazolium cations give salts having low melting points 1-butyl 3-methyl, 1-ethyl 3- methyl imidazolium are most investigated structures18 .
4. EFFECT OF PROPERTIES ON IONIC
LIQUIDS 4.1.
Density In general, ionic liquids are denser than water. The molar mass of the anion significantly affects the overall density of ILs. The [Ms2N] - species have lower densities than the [Tf2N] - salts, 3 in agreement with the fact that the molecular volume of the anion is similar but the mass of the fluorine is greater2 .
4.2. Melting point and crystal structure
The melting point of ILs represents the lower limit of the liquid gap and together with thermal stability defines the interval of temperatures within which it is possible to use the ILs as solvents By examining the properties of series of imidazolium based ionic liquids found that melting point decreases when size and asymmetry of cation increases. Increase in branching of the alkyl chain increases melting point3 .
4.3. Viscosity
A high viscosity may produce a reduction in the rate of many organic reactions and a reduction in the diffusion rate of the redox species. Examining various anion–cation combinations, the increase in viscosity observed on changing selectively the anion or cation has been primarily attributed to an increase in the van der Waals forces. The symmetry of the inorganic anion has sometimes been considered as an additional parameter; viscosity decreases in the order Cl- > [PF6] - > [BF4] - >[Tf2N]- . 4
Most ILs exhibit high thermal stability; the decomposition temperatures reported in the literature are generally >4000C, with minimal vapor pressure below their decomposition temperature the thermal decomposition decreases as the ion hydrophobicity increases. It has been suggested that the stability dependence on the anion is [PF6] - > [Tf2N]- > [BF4] - > halides4 .
4.5. Refractive index
This parameter is related to polarizability/ dipolarity of the medium and the excess molar refraction is used in the least-squares energy relationship of Abraham as a predictor of solute distribution. The values found for [bmim] [X] salts are comparable to those for organic solvent5 .
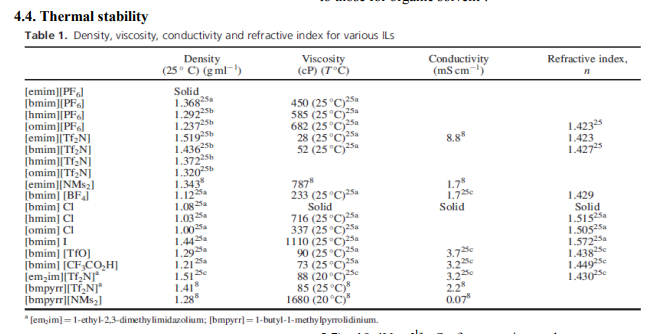
4.6. Surface tension
Surface tension is one of the important properties in multiphase processes. ILs are widely used in catalyzed reactions, carried out under multiphase homogeneous conditions, that are believed to occur at the interface between the IL and the overlying organic phase. In general liquid/air surface tension values for ILs are somewhat higher than those for conventional solvents [(3.3– 5.7) -10-4Ncm-1 ], Surface tension values vary with temperature and both the surface excess entropy and energy are affected by the alkyl chain length, decreasing with increasing length6 .
5. PREPARATION OF SOME IONIC LIQUIDS
5.1. Preparation of Supported Basic Ionic Liquid
A mixture of polystyrene resin and 1- methylimidazole in toluene was refluxed for 24 h. After cooling to room temperature, the reaction mixture was filtered, and the supported ionic liquid 1 (SIL 1) was washed with toluene, HCl, water and methanol, followed by drying under reduced pressure to give SIL1 19 .

5.2. Task-Specific Ionic Liquid, 1, 8- Diazabicyclo [5.4.0] - undec-7-en-8-ium Acetate
Basic task-specified ionic liquid, 1, 8- diazabicyclo [5.4.0]- undec-7-en-8-ium acetate ([DBU] [Ac]) through the neutralization reaction of equal molar amounts of DBU and acetic acid. To a three necked flask was added DBU. Acetic acid was then added drop wise at the temperature of 65 ºC cooled by ice bath. After drop wise addition, the ice bath was removed and the reaction mixture was stirred at room temperature for 24 h. The oil residue was dried in vacuum at 60ºC for 24 h to afford [DBU] [Ac] as a light yellow, viscous liquid20 .

5.3. Synthesis of ammonium cationbased ionic liquids
The aliphatic quaternary ammonium (AQA) cation is a useful cationic component of room temperature ionic liquids, since the salts containing AQA cations and appropriate oxidation resistant anions such as ClO4, BF4 or PF6 are electrochemically stable and may be used as a supporting electrolyte. The asymmetric amide anion (CF3SO2-N-COCF3) K has an excellent ability to lower both the melting points and viscosities of room temperature ionic liquids, combining with the small aliphatic cations21 .
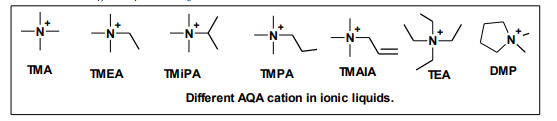
5.4. Synthesis of the functionalized imidazolium ionic liquids
Most of the ionic liquids are based on the 1, 3 dialkyl imidazolium, or 1-alkyl pyridinium ions and also different anions are involved, scientist Davis and Rogers have reported the synthesis of imidazolium salts with urea, thiourea, and thioether groups in one of the N-alkyl substituents22 . Quaternisation of 1-alkyl-1, 2, 4-triazoles at N-4 using polyfluoroalkyl halides under neat reaction conditions at 100–1200C gave the 1-alkyl-4-polyfluoroalkyl-1, 2, 4- triazolium halides [taz] [X]. Metathesis of these polyfluoroalkylated triazolium halides with fluorine-containing anions led to the formation of new 1, 4-alkyl (polyfluoroalkyl)-1, 2, 4-triazolium ionic liquids [R (Rf) taz] [Y].
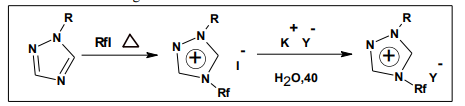
5.5. Synthesis of the chiral ionic liquids
The first reported chiral ionic liquid was 1- butyl-3- methylimidazolium ([BMIM]) lactate III by Seddon et al. in 1999. Chiral ionic liquids are quite attractive for their potential application to chiral discrimination including asymmetric synthesis, optical resolution of racemates chiral pool synthesis23 . Gaumont and co-workers described the synthesis of a new class of chiral ILs (XI, XII) based on thiazolinium salts24. The methodology is outlined in various chiral thiazolinium ILs were obtained by alkylation of chiral thiazoline followed by metathesis of the anion.
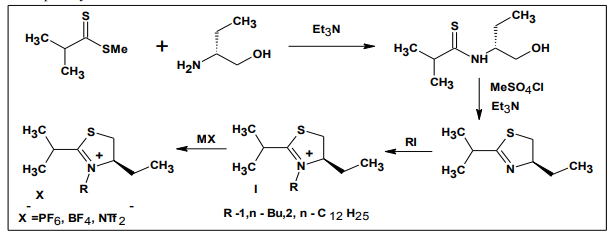
Melting points of these new thiazolinium ILs fell in a range from 1370C to temperatures below 00C. The differences in melting points were due to the different length of the Nalkyl chains and the nature of the counter anions. Unlike the chiral oxazolinium-based ILs, the thiazolinium ILs had good stability under basic and even acidic conditions.
6. APPLICATION OF IONIC LIQUIDS IN NAME REACTIONS
6.1. Aldol condensation reaction
The self condensation reaction of propanol to form 2-methylpent2-enal has been carried out in non coordinating imidazolium ionic liquids. The most selectivity was found for [bmim] [PF6].The reaction of ionic liquid [bmim] [PF6] with proline of different aromatic aldehydes with acetone achieved good results for aldolisation products even for less concentration of the proline used as a catalyst25 .

6.2. Wittig reaction
Wittig reaction is the mainly used in organic synthesis. It is having wider application as synthesis of the alkanes. The ionic solvent [bmim] [BF4] has been used as a medium to carry out Wittig reactions using stabilized ylides allowing easier separation of the alkenes from Ph3PO and also recycling of the solvent. Further the Esteroselectivity was observed in the ionic liquid solvents, similar to that observed in organic solvents7 .
6.3. Friedel crafts reaction
This is one of the reactions used in organic synthesis which involve acylation. It was found that Friedel crafts reaction were carried out by wilkies in an [emim] [Cl]– AlCl3 system27. This was followed by the work of Seddon and Adams, who carried out the acetylation reactions of carbocyclic aromatic compounds with acetyl chloride in acidic compositions of [emim] [Cl]– AlCl3 8 . These reactions worked efficiently giving the stereo electronically favored products. Another interesting development is the use of [BMIM] [chloroaluminate] as Lewis acid catalyst for the Friedel Crafts sulfonylation of benzene and substituted benzenes with TsCl9 .
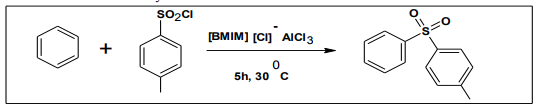
6.4. Diels- alder reaction
It has found that Ionic liquids such as [bmim] [BF4], [bmim] [ClO4], [emim] [CF3SO3], [emim] [NO3] and [emim] [PF6] were demonstrated as effective solvents for Diels–Alder reactions. The reaction between cyclopentadiene and methyl acrylate showed significant rate enhancements, high yields and strong endo selectivity‘s comparable with the best results obtained in conventional solvents10 .
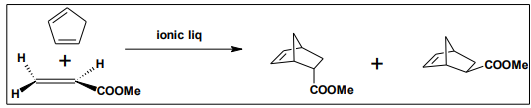
6.5. Heak reaction
The first example of a Heck reaction in ionic liquids was reported for the synthesis of trans-cinnamate by the reaction of bromo benzene with butyl acrylate catalyzed by palladium salts in molten tetra alkyl ammonium and tetra alkyl phosphonium bromide salts.11 The Heck coupling of aryl halides with allylic alcohols catalyzed by PdCl2 in molten tetra alkyl ammonium bromide gave the corresponding B-acrylate carbonyl compounds28

6.6. Suzuki coupling
The Suzuki coupling reaction using pd catalyst with ionic liquids found to be excellent yield and turnover numbers at room temperature 12 . As compared to conventional Suzuki coupling reaction with ionic liquids proceed with high reaction rate. The reaction of bromo benzene with phenyl boronic acid under conventional Suzuki conditions gave 88% yield in 6h(TON, 5h-), and with [bmim][BF4] gave 93% yield in 10min.(TON, 455h-). The use of ultrasound in palladium with [bmim] [BF4] allowed the Suzuki coupling of various aryl halides with phenyl boronic acid to be conducted at room temperatures.
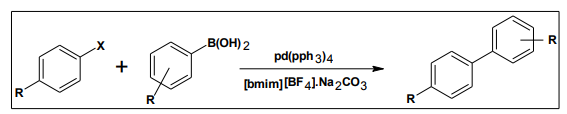
7. APPLICATION OF THE IONIC LIQUIDS IN ANALYTICAL CHEMISTRY
7.1. In MALDI mass spectroscopy
Matrix-assisted laser desorption ionisation (MALDI) mass spectroscopy is one of the soft ionisation methods for analyzing polar, non-volatile and thermally labile bimolecular and synthetic polymers with high molecular weights29, 30. Room temperature ionic liquids with a high solubilising power, negligible vapour pressure, a broad liquid range and the ability to absorb laser light have been designed to use as MALDI matrices. They have found wide applications in peptide and protein quantification, as well as in the analysis of phospholipids. Ionic liquid matrices in MALDI are found to improve the resolving power, reproducibility, and ability to quantify.
7.2. In gas chromatography
In 2007, Han & Armstrong reviewed the role of ILs in separation techniques including GC. Creating GC stationary phases with high thermal stability is very important if IL stationary phases are to compete with commercial GC stationary phases. The GC stationary phages with good thermal stability were prepared by dicationic ionic liquids31. Dicationic ILs with vinyl groups were cross-linked after being coated on a silica capillary to yield GC stationary phases with very high temperature stability and extremely low column bleed32. Also highlighted in the review is the development of chiral GC stationary phases using ILs. A chiral stationary phase based on N, N dimethylphenyl ephedrine, where the IL itself is the chiral selector, resulted in enantiomeric separations for sulfoxides, alcohols, and epoxides33 .
7.3. In liquid-liquid extraction
Recently, several hydrophilic ILs were tested for their ability to extract ethanol from a mixture of ethyl acetate and ethanol34. 1-allyl-3-methylimidazolium chloride, which is used for the extraction and it, can be recycled easily. Recent studies have focused upon penicillin G35 , double-stranded DNA36. The IL trioctylmethylammonium chlorides (TOMAC) more successfully extracted penicillin G than did imidazolium-based ILs, but it was difficult to remove the penicillin from the IL. Thus the ionic liquid has found to be better application in case of the liquid liquid extraction.
7.4. Biocatalysis in ionic liquids
Ionic liquids mainly used in case of the biocatalysis.The use of enzymes in organic solvents rather than in aqueous media greatly enhance their technological applications. Biocatalysis in organic solvents having some disadvantages of reduced activity, selectivity or stability of enzyme as compared to aqueous media. Ionic liquids are used in three different methods in the enzyme systems, namely a) as a co-solvent in aqueous phase, b) as a pure solvent and c) as a two-phase system together with other solvents the use of organic solvents solved the problems. Their use in lipase-catalyzed reactions has increased the solubility of the substrate by 3-fold, and in the N-acetyl-lactosamine synthesis using b-galactosidase, the yield has been doubled by minimizing the side reactions37 . Erbeldinger et al. reported the synthesis of Z-aspartame, catalyzed by thermolysin in [bmim] [PF6], the reaction being carried out in [bmim] [PF6] containing water (5% v/v). The rate of the reaction is comparable to the conventional method38 .
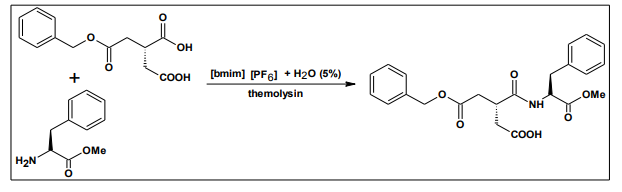
7.5. Other Applications
Ionic Liquid-Derived Blood-Compatible Composite Membranes are used for Kidney Dialysis. In this method Saravanababu Murugesan et. al used room temperature ionic liquids39. In this method a novel heparin- and cellulose-based biocomposite is fabricated by exploiting the enhanced dissolution of polysaccharides in room temperature ionic liquids (RTILs). This represents the first reported example of using a new class of solvents, RTILs, to fabricate blood-compatible biomaterials. The other applications such as Electrochemistry40, Radical polymerization16 , Ionic Polymerization41 and Microwave-Assisted synthesis42 . Hamid Reza Kalhor Et.al in 2009 reported that ionic liquids are also used in inhibition of the amyloid formation43 .
8. TOXICITY OF THE IONIC LIQUIDS
The little data is available on the toxicity of the ionic liquids. Experimental studies have been helpful in establishing general guidelines for the selection of ILs with low potential for toxicity. Many ILs are similar to cationic surfactants, which are known to induce polar narcosis due to their ability to be incorporated into biological membranes many of the imidazolium and pyridinium compounds were more toxic than common high-volume solvents such as acetonitrile, acetone and methanol. Some exceptions include low alkyl chain length quaternary ammonium ILs and those ILs containing choline as the cation, which were relatively nontoxic. Antimicrobial activity increased as the alkyl chain length increased on pyridinium, imidazolium, and quaternary ammonium salts44 . Toxicity was predicted to increase slightly with the number of nitrogen atoms having two aromatic bonds and one single bond. Thus ammonium cations are less toxic than pyridinium cations, which are slightly less toxic than imidazolium cation. The IL toxicity should decrease as the number of negatively charges atoms on the cation increases. Also it was predicted that anions play role in toxicity of the ionic liquids the results indicate that the presence of positively charged atoms in the anion leads to higher toxicity than those systems with a single negative anion atom.
CONCLUSIONS
Rapid growth of interest in application of ILs in medicinal chemistry and analytical chemistry is reasonable in view of their unique properties. Ionic liquids provide chemists with the opportunity of studying reactions in a fascinating new environment. While the fundamental properties of ionic liquids are being unraveled chemists are developing new methods to handle and study them. The use of ILs as ?green chemistry? modifiers is recommended instead of the conventional environmentally harmful agents, which are currently widely employed in analytical practice.
References:
1. Holbrey JD, Reichert WM, Swatloski RP, Broker GA, Pitner WR, Seddon KR, Rogers RD. Green Chem. 2002, 4, 407-13.
2. Pringle JM, Golding J, Baranyai K, Forsyth CM, Deacon GB, Scott JL, MacFarlane DR. Knew. Chem. 2003, 27, 1504-10.
3. (a) Huddleston JG, Visser AE, Reichert WM, Willauer HD, Broker GA, Rogers RD. Green Chem. 2001, 3, 156-164; (b)Dzyuba S, Bartsch RA. ChemPhysChem. 2002, 3, 161–166; (c)Carda-Broch S, Berthold A, Armstrong DW. Anal. Bioanal. Chem. 2003, 375, 191-99.
4. Ngo HL, LeCompte K, Hargens L, McEwen AB. Thermochim. Acta. 2000, 357, 97–102.
5. Cinzia C and Daniela P. J. Phys. Org. Chem. 2005; 18, 275-97.
6. Law G, Watson PR. Langmuir. 2001, 17, 6138-41.
7. Le Boulaire, V. Gre´e, R. Chem. Commun. 2000, 2195.
8. Nara SJ. Harjani JR. Salunkhe MM. J. Org. Chem. 2001; 66, 8616. 9. Fischer T. Sethi T. Welton T. Woolf, J. Tetrahedron Lett.1999, 40, 793. 10. Kaufmann, D. E.; Nouroozianand, M. Henze H. Synlett. 1996, 1091.
11. ) Bouquillion S.Ganchegui B. Estrine B. Henin F. Muzart, J. J. Organomet. Chem. 2001, 634, 153.
12. Karas M. Hillenkemp F. Anal. Chem. 1998, 60, 2299.
13. Erbeldinger M, Mesiano AJ, Russel AJ. Biotechnol. Prog. 2000, 16, 1129.
14. Han X, Armstrong DW, Acc. Chem. Res. 2007, 40, 1079-86.
15. Brinchi L, Germani R, Savelli G. Tetrahedron Lett. 2003; 44, 2027-2029.
16. Wasserscheid P, Welton T (eds). Wiley-VCH: Weinheim. 2003, 69.
17. Xu W, Wang L-M, Nieman R A, Angell C A. J. Phys. Chem. B 2003; 107, 11749-11756.
18. Dyzuba SV, Bartsch R A. Chem. Commun. 2001, 16, 1466-1467.
19. L.F. Xiao, Q.F. Yue, C.G. Xia, L.W. Xu, J. Mol. Catal.A: Chem. 2008, 279, 230.
20. A.G. Ying, L. Liu, G.F. Wua, G. Chen, X.Z. Chen, W.D. Ye, Tetrahedron Lett. 2009, 501, 653.
21. Matsumoto H. Kageyama H. Miyzaki Y. Chem. Commun. 2002, 1726.
22. Visser AE, Swatloski RP, Reichert WM, Mayton R, Sheff S. Wierzbicki A, Davis JH, Rogers, RD. Chem.Commun. 2001, 135. 23. Zhang J, Martin GR, DesMarteau D. Chem. Commun.2003, 2334.
24. Levillain J, Dubant G, Abrunhosa I, Gulea M, Gaumont A-C. Chem Commun. 2003, 2914-2915.
25. Mehnert CP, Dispenziere NC, Cook RA. Chem.Commun. 2002, 1610.
26. Le Boulaire V, Gre´e R. Chem. Commun. 2000, 2195.
27. Earle MJ, Seddon KR, Adams CJ, Roberts G. Chem.Commun. 1998, 2097.
28. Mathew CJ, Smith PJ, Welton T. Chem. Commun.2000, 1249.
29. Cotter RJ. Anal. Chem. 1992, 64, 1027A.
30. Zabet-Moghaddam M, Heinzle E, Tholey A. Rapid Commun. Mass Spectrom. 2004, 18,141-48.
31. Anderson J L, Armstrong D W Anal. Chem. 2005, 77, 6453-62.
32. Berthod A, He L, Armstrong DW. Chromatographia. 2001, 5, 63-68.
33. Ding J, Welton T, Armstrong DW. Anal. Chem. 2004, 76, 6819-22.
34. Matsumoto M, Ohtani T, Kondo K. J. Membr. Sci. 2007, 289, 92-96.
35. Wang J-H, Cheng DH, Chen X-W, Du Z, Fang Z-L. Anal. Chem. 2007, 79, 620-25.
36. (a) Harrison S, MacKenzie SR, Haddleton DR, Polym Prepr (Am Chem Soc Div Polym Chem. 2002, 43(2), 883-84. (b) Harrison S, MacKenzie SR, Haddleton DR. Chem Commun. 2002, 2850-51.
37. Seda Keskin e, J. of Supercritical Fluids. 2007, 43, 150-80. 38. Jain N, Kumar A, Chauhan S and Chauhan S. M. S, Tetrahedron. 2005, 61, 1015-60.
39. Saravanababu Murugesan , Wiley InterScience. 2005, 298-304.
40. Lu X, Hu J, Yao X, Wang Z, Li J. Biomacromolecules. 2006, 7, 975-80.
41. Damlin P, Kvarnstrom C, Ivaska A. J Electroanal Chem. 2004, 570, 113–22.
42. Hua Zhao , Zhiyan Song, Janet V. Cowins and Olarongbe Olubajo, Int. J. Mol. Sci. 2008, 9, 33-44.
43. Hamid Reza Kalhor, Biomacromolecules. 2009, 10, 2468- 75.
44. Hajipour AR and. Rafiee F, J. Iran. Chem. Soc. 6, December 2009, 647-78.
|