IJCRR - 3(10), October, 2011
Pages: 19-27
Print Article
Download XML Download PDF
TOLL LIKE RECEPTORS: THE IMMUNOMODULATORY AGENTS AND NOVEL TARGET OF IMMUNOTHERAPEUTIC RESEARCH
Author: L.K. Dwivedi, Desh D. Singh, Rambir Singh
Category: Healthcare
Abstract:Toll-like Receptors (TLRs), the evolutionarily conserved molecules are identified as the pattern
recognition receptors (PRRs) in vertebrates and invertebrates which recognize the pathogenassociated
molecular patterns (PAMPs). The TLRs signaling is via interactions with adaptor
proteins including MyD88 and toll receptor associated activator of interferon (TRIF). They&
directly detect the pathogen invasion and induce either immuno-stimulatory or
immunomodulatory biological response. This ability of TLRs to modulate the immune system
has been taken in concern in recent studies to develop an adaptive immunotherapy against
cancer and several other neurological disorders. Moreover, to trace their auxiliary therapeutic
effects, the TLR agonists are now undergoing the extensive clinical investigation. This review
discusses the therapeutic potential of TLRs as Immunostimulators and Immunomodulators.
Alongside, the association of TLRs with autoimmune responses and human diseases is also
explored.
Keywords: TLR, PRS, PAMP, Targeted therapy, autoimmune response
Full Text:
INTRODUCTION
The Toll-like receptors are so named because of their similarity to the Drosophila Toll receptor (Leimaitare et al., 1996). They recognize the pathogenassociated unique molecules such as the bacterial cell wall components peptidoglycan (TLR2) and lipopolysaccharide (TLR4) (Zhang et al., 2004). The dsRNA, ssRNA and nonmethylated Cytosine-Guanosine (CpG) DNA are also identified by TLR3, TLR7 and TLR9 respectively (Akira and Takeda, 2004; Takeda et al., 2003) (Fig.1). As a consequence, after activation of TLRs by said ligands the different cytokine and chemokines are produced which can further initiate the local inflammatory response to provide a first-line defense against pathogen invasion. The TLRs are mostly located on antigen presenting cells. Each of them, with the exception of TLR3, signals through the MyD88 (myeloid differentiation88 gene) dependent pathway, initiated by the MyD88 adaptor protein (Broad et al., 2007). The MyD88 recruitment at TLRs instigates the formation of Imterleukin-1 (IL-1) receptor associated kinase (IRAK) complex (Fig.2). Four members of this family have been identified: IRAK-1, IRAK-2, IRAK-4 and IRAK-M (Björkbacka, 2006). Knockout mice for IRAK-4 had completely abolished responses to TLR2, TLR3, TLR4 and TLR9 showing that this molecule is essential for TLR signaling (Pasterkamp et al., 2004). Also, the MyD88 deficient cells show the increased expression of costimulatory molecules, such as CD80 and CD86 to induce dendritic cell maturation in the absence of MyD88 dependent pathway. Formation of IRAK complex further result in phosphorylation of IKKa/b, activation of the transcription factors NFkB, IRF1, and IRF7, and generation of the proinflammatory cytokines IL-6 and TNFα and others (Takeuchi et al., 200; Brenda et al., 2008). While TLR3 signals through the MyD88 independent pathway, initiated by the TRIF adaptor molecule (Broad et al., 2007) which unlike to others initiates the phosphorylation of IKKe and activates the transcription factors IRF3 and IRF7 to generate the anti-viral molecules such as Interferon β (IFNβ) (Brenda et al., 2008). Amongst TLRs, only TLR4 can utilize either of these pathways (Fig-2). The TLRs are proved to be a key link between obesity, insulin resistance, and cardiovascular disease. Pharmacologic approaches to modify the activity of the TLRs may therefore have favorable influences on the atherosclerotic disease process and other cardiovascular disorders (Boekholdt et al., 2008).
TLRs AND TH RESPONSE
Since, TLRs are widely expressed on immune cells such as Dendritic Cells (DCs), monocytes, mast cells, neutrophils, B cells, endothelial cells and fibroblasts therefore; they activate these cells to produce IL-12 and IFN-α to mount the Th1 responses. However, apart from the professional antigen presenting cells (APCs), TLRs are also found expressed on non-immune cells such as mesangial, astrocytes, uterine epithelial and fibroblasts cells (Lutz and Schuler 2002). Role of TLRs in the development of classic Th2 responses is still debatable. Though, highly purified P. gingivalis LPS, a putative TLR2 ligand (Methe et al., 2005) Pam-3-cys, a synthetic TLR2 ligand and Schistosomal Egg Antigen (SEA) have been shown to induce the Th2 responses (Sakata et al., 2007).
TLRs AS IMMUNOSTIMULATORS TLRs
being organism-wide sensor system play a frontline effector role in host defense. Hitherto, many TLR agonists have been developed to be used as vaccine adjuvants to stimulate and enhance the antigen specific memory response and circumvent the booster burden. Recently, the utilization of TLR agonists against neural cancer has been an emerging strategy to stimulate the tumor specific natural adaptive anti-tumor immune response. In this approach, specific timorous area is only targeted along with protection of normal brain structures. Several TLR agonists have been investigated in this regard. One particular candidate the Imiquimod has got most attention in cancer immunotherapy. It is a synthetic TLR7 agonist that has been given the Food and Drug Administration (FDA) approval as a topical treatment for Herpes Sarcoma Virus (HSV)-2 lesions (Prins et al., 2006). In a recent study, the topical application of Imiquimod has been shown to decrease intracranial tumor burden in a malignant melanomic mouse model (Prins et al., 2006). It is believed that the said effect was shown due to prolonged existence of antigen-pulsed dendritic cells and improved CD8+ priming. However, a prolonged Imiquimod treatment has also been reported the increased hemorrhaging and inflammation-induced mortality. Lipopolysaccharide (LPS), a potent agonist of TLR4 has also been investigated for its anti-tumor immune efficacy. In a study by Won et al (2003), the LPS have been shown to decrease the mean tumor mass of primary subcutaneous Glioblastoma Multiforme (GM) tumors in induced mouse model. Supportively, the LPS administered mice bearing intracranial GM tumors have been shown the increased survival thanTLR4 knockout mice of the same group. The observations suggested that the LPS cytotoxicity is however insufficient to clear the tumors but certainly may act as a tumor-specific immune adjuvant (Chicoine et al., 2007). The chemotherapeutic use of one more agonist of TLR9, the nonmethylated CpG oligodeoxynucleotides have also got attraction and shown the great promise. It has been demonstrated that the intratumoral CpG administration in mice and rats with subcutaneous and intracranial neuroblastoma tumors increases the survival time and protects against secondary tumor challenge (Carpentier et al., 1999). It induces the apoptosis of intracranial glioblastoma cells (El Andaloussi et al., 2006). TLR9 is chiefly expressed by glial cells in mice but restricted to the B cells and plasmacytoid dendritic cells in healthy humans subjects (Iwasaki et al., 2004). A Phase II clinical trial is in progress to explore the therapeutic potential of said ligand into generation of a specific anti-tumor immune response in patients with brain tumors.
TLRs AS IMMUNOMODULATORS
In addition to immunostimulation, immunosuppressive properties of TLRs are also exploited to develop immunotherapy against neurological disorders. Such immunosuppressive therapies are based on the phenomena of ?tolerance?, where moderate pre-exposure of deleterious stimulus potentiate the animals to show the tolerance against subsequent intensive exposure of the same. For instance, the mild pretreatment of LPS can protect an animal from detrimental effects during subsequent higher exposure of the same. Supportively, a study by Tasaki et al (1997) demonstrated that the systemic administration of low dose LPS subsequently render the tolerance in hypertensive rats to ischemic brain damage induced by middle cerebral artery occlusion (MCAO). The mechanism behind is believed that the inflammatory response induced during pretreatment of LPS enhances the blood perfusion towards ischemic area at the time of ischemia and afterwards (Kunz et al., 2007; Dawson et al., 1997). Moreover, LPS pretreatment has also been reported to protect brain against cytotoxic effects of TNFα after cerebral ischemia (Rosenwieg et al., 2004). It is observed that the LPS tolerant Cells possess deficient ability to generate TNFα in response to TLR4 activation. These cells unlike to naïve cells, do not recruit MyD88 to TLR4, and fails to activate IRAK-1and NFkB thereby leading to blockage in TNFα expression (Medvedev et al., 2002). Since, Dendritic Cells (DC) contain TLR9 thus it is observed that after exposure of DCs from TLR9 agonists (with or without antigen), various pro-inflammatory cytokines and chemokines were secreted which further activated and some time suppressed (caused due to specific cytokine viz. IL-6) to the effector T cells (Th1 and/or CTL cells) response (Pasare and Medzhitov, 2003) (Fig 3).
ROLE OF TLRs’ IN AUTOIMMUNE RESPONSE
The autoimmunity is a consequence of unbridled proliferation of auto-reactive immune cells along with defects in the maintenance of tolerance (Yamada et al., 2005). Several evidences have now established that TLRs contribute to the development of autoimmunity (Suzuki et al., 2002). The virus induced IFN-α, whose effect can be mediated via TLRs 3, 7 and 9, contribute significantly in the pathogenesis of type I diabetes mellitus and Systemic Lupus Erythematosus (Kobayashi et al., 2002). Moreover, viral dsRNA (double stranded RNA) can bind to TLR3 and activate NF-κB which induces the formation of apoptotic bodies that further promotes plasmacytoid DCs to produce IFN-α (Kawai, 2001). B cells as professional antigen presenting cells express both TLR7 and TLR9. Out of which, TLR 9 are reported to break the tolerance of auto-reactive B cells through binding to hypomethylated CpG-containing DNAs, which are present in the mammalian DNA itself (Doyle et al., 2002). These regions, which may be the derivatives of apoptotic or necrotic cells, form a complex with anti-DNA IgG autoantibodies and stimulate B cells via BCR and TLR 9 (Kaisho et al., 2001).
POSSIBLE ROLE OF TLRs IN HUMAN DISEASES TLRs
being active immunomediators recognize the various endogenous proteins that are often released in response to stress or tissue damage and variably found at the sites of chronic inflammation. These may be heat shock proteins (hsp) 60, 70, 96, extra domain A-containing fibronectin fragments, hyaluronan fragments, fibrinogen, β-defensins, oxidized lowdensity lipoprotein and heparan sulfate
CONCLUSION and FUTURE PERSPECTIVES Above discussion has demonstrated the TLRs as an immunomodulatory agent who can generate the protection against cancer and suppress the damaging inflammatory responses. Captivatingly, the ability of TLRs to activate tumor specific lymphocytes has been translated into antitumor immunotherapy in recent studies.
Also, the TLR agonists based immunostimulation has been proven its success in treating the neurological disorders. Though, owing to systemic cytokine induction, the systemic use of TLR agonists may be restricted by doselimiting toxicities but combinatorial approaches with conventional therapies such as chemotherapy may be promising. However, pharmacological interventions using TLRs antagonists are in infancy but hold much promise for future success. Therefore, with increasing evidences of active role in initiation and maintenance of immune response, the TLRs can be an attractive drug targets for regulating the immune terror
ACKNOWLEDGEMENT
Authors acknowledge the kind support of Dr. Vinod Singh, Associate Professor, Department of Microbiology, Barkatullah University, Bhopal, MP, India during preparation of the manuscript.
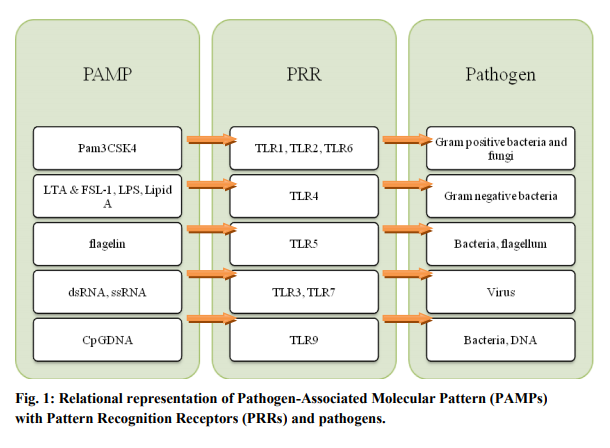
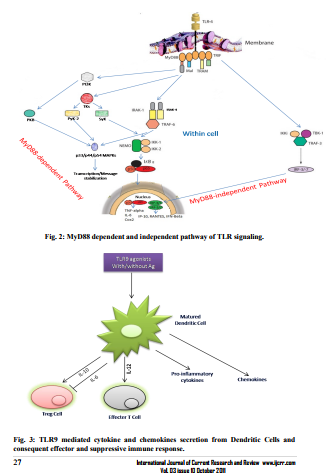
References:
REFERENCES
1. Akira, S., and K. Takeda. 2004. Tolllike receptor signaling. Nat Rev Immunol., 4: 499-511.
2. Anders, H.J., D. Zecher, R.D. Pawar and P.S. Patole. 2005. Molecular mechanisms of autoimmunity triggered by microbial infection. Arthritis Res and Ther.,7: 215-224.
3. Björkbacka, H. 2006. Multiple roles of toll-like receptor signaling in atherosclerosis. Curr Opin Lipidol., 17: 527-533.
4. Boekholdt, S.M., R.J. Winter, J. Kastelein. 2008. Inhibition of lipoproteinassociated phospholipase activity by darapladib: shifting gears in cardiovascular drug development: are antiinflammatory drugs the next frontier. Circulation, 118: 1120 –1122.
5. Brenda J. Marsh, B.S. and Mary P. Stenzel-Poore. 2008. Toll-like Receptors: Novel Pharmacological Targets for the Treatment of Neurological Diseases. Curr Opin Pharmacol., 8(1): 8–13
6. Broad, A., J.A. Kirby and D.E. Jones. 2007. Toll-like receptor interactions: tolerance of MyD88-dependent cytokines but enhancement of MyD88- independent interferon-beta production. Immunology, 120: 103–111.
7. Carpentier, A.F., L. Chen, F. Maltonti and J.Y. Delattre. 1999. Oligodeoxynucleotides containing CpG motifs can induce rejection of a neuroblastoma in mice. Cancer Res., 59: 5429–5432
8. Chicoine, M.R., M. Zahner, E.K. Won, R.R. Kalra, T. Kitamura, A. Perry and R. Higashikubo. 2007. The in vivo antitumoral effects of lipopolysaccharide against glioblastoma multiforme are mediated in part by Toll-like receptor 4. Neurosurgery, 60: 372–380.
9. Dawson, D.A., K. Furuya, J. Gotoh, Y. Nakao and J.M. Hallenbeck. 1999. Cerebrovascular hemodynamics and ischemic tolerance: lipopolysaccharideinduced resistance to focal cerebral ischemia is not due to changes in severity of the initial ischemic insult, but is associated with preservation of microvascular perfusion. J. Cereb. Blood Flow Metab., 19: 616–623
10. Doherty, T.M., E.A. Fisher, M. Arditi. 2006. TLR signaling and trapped vascular dendritic cells in the development of atherosclerosis. Trends Immunol., 27: 222-227.
11. Doyle, S., S. Vaidya, R. O‘Connell, H. Dadgostar and P. Dempsey et al., 2002. IRF3 mediates a TLR3/TLR4 specific antiviral gene programme. Immunity, 17: 251-63.
12. El Andaloussi, A., A.M. Sonabend, Y. Han and M.S. Lesniak. 2006. Stimulation of TLR9 with CpG ODN enhances apoptosis of glioma and prolongs the survival of mice with experimental brain tumors. Glia, 54: 526–535.
13. Iwasaki, A. and R. Medzhitov. 2004. Toll-like receptor control of the adaptive immune responses. Nat. Immunol., 5: 987–995.
14. Kaisho, T., O. Takeuchi, T. Kawai, K. Hoshino and S. Akira. 2001. Endotoxin induced maturation of MyD88 deficient dendritic cells. J. Immunol., 166: 5688-5694.
15. Kawai, T., O. Takeuchi, T. Fujita, J. Inoue, P.F. Mühlradt and S. Sato. 2001. Lipopolysaccharide Stimulates the MyD88-Independent Pathway and Results in Activation of IFNRegulatory Factor 3 and the Expression of a Subset of LipopolysaccharideInducible Genes. J. Immunol., 167: 5887 –5894.
16. Kawakami, A., M. Osaka, M. Aikawa, S. Uematsu and S. Akira et al., 2008. Toll-like 2 receptor mediates apolipoprotein CIII-induced monocyte activation. Circ. Res., 103: 1402–1409.
17. Kobayashi, K., L.D. Hernandez, J.E. Galan, C.A. Janeway, R.A. Medzhitov Rand Flavell. 2002. IRAK-M is a negative regulator of Toll-like receptor signaling. Cell, 110: 191-202.
18. Kunz, A., L. Park, T. Abe, E.F. Gallo, J. Anrather, P. Zhou and C. Iadecola. 2007. Neurovascular protection by ischemic tolerance: role of nitric oxide and reactive oxygen species. J Neurosci., 27: 7083–7093.
19. Leadbetter, E.A., I.R. Rifkin, A.M. Hohlbaum, B.C. Beaudette, M.J. Shlomchik and A. Marshak-Rothstein. 2002. Chromatin-IgG complexes activate B cells by dual engagement of IgM and Toll-like receptors. Nature, 416: 603–607.
20. Leimaitare, B., E. Nicolas, L. Michaut, J-M Reichhart and J.A. Hoffman. 1996. The dorsoventral regulatory gene cassette spatzle/Toll/ cactus controls the potent antifungal response in Drosophila adults. Cell, 86: 973-983.
21. Lutz, M.B., and G. Schuler. 2002. Immature, semi-mature and fully mature dendritic cells: which signal induces tolerance and autoimmunity?. Trends Immunol., 23: 445-449.
22. Lynn, M., D.P. Rossignol, J.L. Wheeler, R.J. Kao and C.A. Perdomo et al., 2003. Blocking of responses to endotoxin by E5564 in healthy volunteers with experimental endotoxemia. J. Infect. Dis., 187: 631- 639.
23. Medvedev., A.E., A. Lentschat, L.M. Wahl, D.T. Golenbock and S.N. Vogel. 2002. Dysregulation of LPS-induced Toll-like receptor 4-MyD88 complex formation and IL-1 receptor-associated kinase1 activation in endotoxin-tolerant cells. J Immunol., 169: 5209–5216
24. Methe, H., J.O. Kim, S. Kofler, M. Weis, M. Nabauer and J. Koglin. 2005. Expansion of circulating toll-like receptor 4-positive monocytes in patients with acute coronary syndrome. Circulation, 111: 2654-2661.
25. Pasare, C. and R. Medzhitov. 2003. Toll pathway-dependent blockade of CD4+CD25+ T cell-mediated suppression by dendritic cells. Science, 299(5609): 1033-1036
26. Pasterkamp, G., J.K. van Keulen, D.P.V. de Kleijn. 2004. Role of tolllike receptor 4 in the initiation and progression of atherosclerotic disease. Eur. J. Clin. Invest., 34: 328-334.
27. Prins, R.M., N. Craft, K.W. Bruhn, H. Khan-Farooqi, R.C. Koya, R. Stripecke, J.F. Miller and L.M. Liau. 2006. The TLR-7 agonist, imiquimod, enhances dendritic cell survival and promotes tumor antigen-specific T cell priming: relation to central nervous system antitumor immunity. J. Immunol., 176: 157–164
28. Rosenzweig, H.L., N.S. Lessov, D.C. Henshall, M. Minami, R.P. Simon, M.P. Stenzel-Poore. 2004. Endotoxin preconditioning prevents the cellular inflammatory response during ischemic neuroprotection in mice. Stroke, 35: 2576–2581
29. Sakata. Y., J.W. Dong, J.G. Vallejo, C.H. Huang and J.S. Baker et al., 2007. Toll-like receptor 2 modulates left ventricular function following ischemia-reperfusion injury. Am. J. Physiol. Heart Circ. Physiol., 292: H503-H509.
30. Seibl, R., D. Kyburz, R.P. Lauener, S. Gay. 2004. Pattern recognition receptors and their involvement in the pathogenesis of arthritis. Curr. Opin. Rheumatol., 16: 411-418.
31. Steinman, R.M. 2006. Linking innate to adaptive immunity through dendritic cells. ovartis Found Symposium, 279: 101-109.
32. Suzuki, N., S. Suzuki, G.S. Duncan, D.G. Millar and T. Wada et al., 2002. Severe impairment of interleukin-1 and toll like receptor signaling in mice lacking IRAK-4. Nature, 416: 750-756.
33. Takeda, K., T. Kaisho and S. Akira. 2003. Toll-like receptors. Annu. Rev. Immunol., 21: 335-376.
34. Takeuchi, O., T. Kawai, P.F. Muhlradt, M. Morr, J.D. Raddolf and A. Zychlinsky. 2001. Discrimination of bacterial lipoproteins by Toll-like receptor 6. Int. Immunol., 13: 933-940.
35. Tasaki, K., C.A. Ruetzler, T. Ohtsuki, D. Martin, H. Nawashiro, J.M. Hallenbeck. 1997. Lipopolysaccharide pretreatment induces resistance against subsequent focal cerebral ischemic damage in spontaneously hypertensive rats. Brain Res.,748: 267–270
36. Won, E.K., M.C. Zahner, E.A. Grant, P. Gore and M.R. Chicoine. 2003. Analysis of the antitumoral mechanisms of lipopolysaccharide against glioblastoma multiforme. Anticancer Drugs, 14: 457–466.
37. Yamada, M., T. Ichikawa, M. Ii, M. Sunamoto, K. Itoh, N. Tamura and T. Kitazaki. 2005. Discovery of novel and potent small-molecule inhibitors of NO and cytokine production as antisepsis agents: synthesis and biological activity of alkyl 6-(N-substituted sulfamoyl) cyclohex-1-ene-1- carboxylate. J. Med. Chem., 48: 7457- 7467.
38. Zhang, D., G. Zhang, M.S. Hayden, M.B. Greenblatt, C. Bussey, R.A. Flavel. 2004. A toll like receptor that prevents uropathogenic bacteria. Science, 303: 1522-1526.
|