IJCRR - 4(6), March, 2012
Pages: 63-73
Print Article
Download XML Download PDF
COMPARISON OF WATER SAVINGS OF PADDY RICE UNDER SYSTEM OF RICE INTENSIFICATION (SRI)
GROWING RICE IN MWEA, KENYA
Author: J.A. Ndiiri, B.M. Mati, P.G. Home, B. Odongo, N. Uphoff
Category: Technology
Abstract:Rice is the greatest consumer of water among all crops and uses about 80% of the total irrigated freshwater resources. The high demand for rice in Kenya due to urbanization has led to increases in price. In Mwea, rice is grown under continuous flooding. This system of rice production depends on a continuous supply of water for irrigation and soils with high water holding capacities yet, the main rice growing season coincides with the low rainfall season. Thus, water rationing during this period is inevitable. To be able to meet the growing demand with the depreciating water resources sustainably, new innovative ways of rice crop production are needed. System of Rice Intensification (SRI) is a new innovation that offers an opportunity to reduce water demand accompanied by yield increase of rice. Field experiments were conducted in 2010/2011 at Mwea Irrigation Agricultural Development (MIAD) of Mwea Irrigation Scheme (MIS) during the main growing season (August 2010- January 2011) to assess the effects on water savings for three varieties of rice grown under SRI versus CF. The results showed that SRI gave the highest savings on water, yields hence water productivity for all the three varieties. Yield increased by 0.6t/ha, 2t/ha and 1.5t/ha while water savings were 2528m3/ha, 2268m3/ha and 2846m3/ha for the Basmati 370, BW 196 and IR 2793-80-1 varieties, respectively. Similarly, calculations showed water productivity (kilograms of rice per cubic meter of irrigation water supplied) averaging 120% higher for the three varieties under SRI management (2.16 kg/m3 vs. 0.98 kg/m3).
Keywords: SRI, Rice, Mwea, Water savings, Water productivity
Full Text:
INTRODUCTION
Water available for agriculture is diminishing due to rapid population growth and climate change along with rising demand for food. This is especially true for rice due to urbanization, which has led to an upward shift in demand for rice worldwide as people change their eating habits (Mishra, 2009). Increased rice supply, on the other hand, is constrained due to lack of sufficient water availability as this crop is the largest consumer of water in the agricultural sector (Bera, 2009; Mishra, 2009; Prasad, 2009; Prasad and Ravindra, 2009; Thakur et al., 2011). Rice production in Kenya is based mostly on the conventional practice of continuously flooding the paddy fields (Republic of Kenya, 2008). This method is not sustainable due to the already existing competition for water among farmers within and outside the scheme (Mati et al., 2011). Thus, innovative ways for efficient use of water need to be put in place to ensure sustainable rice production (Bouman et al., 2005; Mati and Nyamai, 2009; Mishra, 2009). The System of Rice Intensification (SRI), developed in Madagascar over 25 years ago (Laulani?, 1993; Sarath and Thilak, 2004), offers this opportunity to improve food security through increased rice productivity by changing the management of plants, soil, water and nutrients while reducing external inputs like fertilizers and herbicides (Berkelaar, 2001; Thakur et al., 2009; Uphoff, 2003; Vermeule, 2009). The system proposes the use of single, very young seedlings with wider spacing, intermittent wetting and drying, use of a mechanical weeder which also aerates the soil, and enhanced soil organic matter (Uphoff et al., 2009). All these practices are aimed at improving the productivity of rice grown in paddies through healthier, more productive soil and plants by supporting greater root growth and by nurturing the abundance and diversity of soil organisms (World Bank Institute, 2008; Stoop et al., 2002). Previous research has shown yield increases of between 50- 100% while irrigation water inputs can be reduced by between 25% and 50% with SRI (Bera, 2009; Berkelaar, 2001; McDonald et al., 2006; Sarath and Thilak, 2004; WBI, 2008). However, little is known about SRI and the impact of its adoption on water savings in Mwea scheme and Kenya as a whole. This study investigated whether SRI practices, particularly transplanting quickly one young seedling per hill, alternate wetting and drying and wider spacing could have significant effects on plant growth and subsequently on water productivity. A detailed comparison of the performance of rice plants grown under SRI and under conventional management practices for three varieties is presented here. Soil and climatic conditions, fertilization and weeding method were the same for both sets of trial.
MATERIALS AND METHODS
Experimental site and soil
This field experiment was conducted in 2010/2011 at the Mwea Irrigation Scheme in Kenya. The experimental site is situated between latitudes 37°13‘E and 37°30‘E and longitudes 0°32‘S and 0°46‘S. The region is classified as tropical with a semi-arid climate, having an annual mean air temperature of 23-25°C with about 10°C difference between the minimum temperatures in June/July and the maximum temperatures in October/March. Annual mean precipitation is 950 mm, with annual sunshine of 2485 h. Land preparation for both CF and SRI was standard wet tillage and harrowing. This was done by first flooding the paddies for three days then paddled to soften and mix the mud as illustrated in Wanjogu et al., 1995. For nursery establishment, germinated seeds for SRI practice were broadcasted on raised beds on 31 August 2010 while those for the conventional practice were broadcasted on 11 August 2010. The soils have been classified previously as Vertisols (Sombroek et al., 1982). The topsoil contained 0.014% available N, 29 ppm available P, and 0.042 meq/100g available K, 1.13% organic carbon, and had a pH value of 6.3 at the start of the experiment. Experimental design and treatments The experimental design was a randomized split-plot design with three replications and subplot sizes of 3m by 3m. In the main plots, rice was grown under the two alternative crop management systems (treatments) of SRI and conventional practices with continuous flooding (CF). Three rice varieties (Basmati 370, BW 370 and IR 2793-80-1) were grown on the plots, with three replications each. Basmati 370 is an aromatic, low tillering, and shortduration, 120 days; BW 196 is a long duration of 150 days and considered high tillering while IR 2793-80-1 is mediumlong duration of 140 days and medium yielding - close to BW 196. Each plot was surrounded by consolidated bunds and lined with plastic sheets installed to 0.3m deep to prevent seepage and nutrient diffusion among plots, followed by 1m wide channels for irrigation. The spacing was 20cm by 20cm for SRI practice and 10cm by 10cm for CF practice. Crop management and irrigation The nursery was adjacent to the main field so that transplanting could be performed quickly to minimize injury to the young plants (WBI, 2008). Seedlings were transplanted on 8 September with 8-dayold seedlings at a rate of one seedling per hill for SRI. At 8 days old, seedlings were still in their second phyllochron as recommended for SRI practice (Stoop et al., 2002). For the CF practice, 28-day-old seedlings were transplanted at a rate of three seedlings per hill. This is the conventional way of growing rice in the scheme. The difference in plant populations was thus 25 compared with 400 per square meter. Both sets of treatments received the same basal fertilizer supply of 125kg/ha DiAmmonium Phosphate (DAP) and 62kg/ha Mulate of Potash (MoP) 1 day before transplanting. All plots received an additional 125kg/ha of Sulphate of Ammonia (SA) 10, 30 and 60 days after transplanting (DAT) according to Wanjogu et al., 1995. No herbicide, insecticide or chemical disease control measures were used. The CF treatments were continuously flooded with water to a depth of 5cm except at the end of the tillering stage when the depth was reduced to 3 cm. The SRI plots were kept saturated at the first week after transplanting. After that and up to panicle initiation stage, plots were maintained with a thin layer (2 cm) of standing water for 2 days and without standing water for 5 days before reirrigation with the river water. At this stage, the cracks ranged between 1-1.5 cm and the moisture content of the soil at 10 cm depth was 40% while that at 20 cm depth was 80%. A rice growth staging system by Wanjogu et al., 1995 was followed to describe the rice growth stages. Climate data and water measurements Data on daily rainfall, pan evaporation, wind speed, daily minimum (Tmin) and maximum temperature (Tmax) were collected from the weather station (Table 1) at the research farm located 500 m away from experimental plots. Water was supplied through a concrete channel to a plot channel and subsequently to the plots. A trapezoidal Parshall flume was installed at the gate provided for each plot during the construction of bunds for the purpose of supplying and measuring water for both practices. However, for the SRI plots, water measurement was made during irrigation and when draining excess water. Water measurement for the CF plots was made only during irrigation. The amount of water applied was estimated by reading both water height and time taken for the water to flow through the Parshall flume and into the plot to the required level, and then converted to the volume of water required for the cropping season (Herschy, 1995; ASTM D1941-91). Each plot was irrigated separately. All plots were drained at 14 days before harvest. Water productivity was estimated as grain yield divided by total water supplied into the plot (rainfall and applied) (Boumann and Tuong, 2000) and expressed as kgm3 . The SRI plots were weeded four times, while CF plots were weeded three times during the growing season. Manual weeding, where weeds were uprooted, was used in both practices since the rotary weeder was not available at the time of the trial. Assessing root dry weight Three hills from each replicate were randomly selected at the early-ripening stage of each variety for collection of root samples. This was done using an auger of 10 cm diameter to remove soil of 20 cm deep along with the hill (Kawata and Katano 1976). A uniform soil volume (1571 cm3 ) was excavated to collect root samples from all the treatments. Roots were carefully washed and dry weight measured (Yoshida 1981). Root volume was measured by the water displacement method of putting all roots in a measuring cylinder and getting the displaced water volume. Measurement of yield and yield components Yields are normally 3-5 t/ha, 7-9 t/ha and 9-11 t/ha for Basmati 370, IR 2793-80-1 and BW 196 varieties, respectively (MIAD Manager, personal comm. 2010). Harvesting for Basmati 370, BW 196 and IR 2793-80-1 under SRI was done after 135 days, 151 days, and 135 days, respectively, while plots under CF were harvested after 141, 172 and 156 days, respectively, giving differences of 6, 21 and 21 days, respectively. All plants in an area of 3m by 3 m for each replicate were harvested for determination of yield per unit area, and grain yield was adjusted to 14.5% seed moisture content using the following equation: Yield in t/ha = [(100- MC)/86*GW*10000]/Plot area Where; MC= Moisture Content after drying, GW= Grain Weight per plot area Harvest Index (HI) was calculated by dividing dry grain yield by the total dry weight of aboveground parts (Thakur et al., 2009). Average tiller number and panicle number were determined from the crop harvested from 1 m2 area per replication. Panicle length, number of grains per panicle, and number of filled grains were measured for each panicle individually harvested from the sample area. The per cent of ripened grains was calculated by dividing the number of filled grains by the number of total grains. Statistical analysis All data were analyzed statistically using analysis of variance (ANOVA) technique as applicable to split-plot design (Gomez and Gomez 1984). The significance of the treatment effect was determined using Ftest; and to determine the significance of the difference between two treatment means, least significant difference (LSD) was estimated at performance of rice at 5% probability level. If the LSD was less than the difference in means between two treatments, then the two treatments were significantly different and vice versa.
RESULTS Grain yield and yield components The System of Rice Intensification plots produced significantly (P=0.026) larger grain yield for all varieties (26% on average) than CF plots. The long-duration and high-yielding BW 196 variety had the highest percentage increase (51%), followed by the mid-duration IR 2793-80-1 at 16% and finally the short-duration at Basmati 370 at 11% (table 2). Among the yield components, grains per panicle, grain-filling percentage, and grain weight were significantly (P < 0.05) affected by cultivation practice and variety (Table 2). IR had the highest number of grains per panicle, followed by Basmati 370 and lastly BW 196. However, SRI panicles had significantly lesser (P=0.85) number of filled grains than CF panicles, although significantly higher (0.006) grain weight than CF. Thus, most of the percentage increase in grain yield and hence in net income is a result of higher grain weight. Overall, SRI plots had significant improvement in various yield components compared with CF plots. Root dry weight The results of root dry weight showed a significant (P= 0.042) improvement in root growth in the SRI plants of all varieties (Table 5). Basmati 370 and BW 196 root dry weight under SRI practice was more than double that of CF practice. However, on an area basis, the root dry weight for IR 2793-80-1 variety was more in CF than SRI practice. Water productivity and water savings During the cropping season, rainfall received was 346.4 mm. However, the SRI plots were drained leaving 61.3 mm, 69.6 mm and 61.3 mm for the Basmati 370, BW 196 and IR 2793-80-1, respectively. Because the rice varieties took different durations, the rainfall amount utilized by the varieties was different. It was 282.1 mm, 346.4 mm and 264.4 mm for Basmati 370, BW 196 and IR 2793-80-1, respectively, under CF practice. There was significant water savings with SRI compared to CF (Table 4). BW 196, the long-duration variety, had the highest consumption, followed by IR 2793-80-1, which was close to Basmati 370. However, IR 2793-80-1 had the highest water savings in both seasons. SRI demonstrated significantly higher water productivity (1.72 kg/m3 , 1.42 kg/m3 and 3.35 kg/m3 for Basmati 370, BW 196 and IR 2793-80-1) compared to CF with 0.89 kg/m3 , 0.61 kg/m3 and 1.54 kg/m3 for the varieties respectively. Average water productivity for the three varieties under SRI management was 2.16 kg/m3 , 120% more than the 0.98 kg/m3 average under conventional management. DISCUSSION System of Rice Intensification (SRI) is an effective water-saving methodology. The objective of the evaluation in this study was to assess the impact of SRI practices on yields and water productivity of paddy rice. The results showed that for all varieties, using SRI methods increased yields and reduced water use thus increasing water productivity. The reduction in water use under SRI practice ranged between 26%-31%. Water productivity was much higher for SRI practice than CF, doubling the grain produced per amount of water input (table 4). Clearly, the rice plants responded better to alternate wetting and drying of the soil compared to conventional CF. Previous studies have shown that yield increase with SRI practices involves some improvement in nutrient availability. Inubushi and Wada (1987) found that drying and rewetting Japanese soils not only generated or enlarged a nutrient pool that mineralized rapidly according to first-order kinetics, but also increased the size of a more stable nitrogen pool which mineralized more slowly. This could be explained by an increase in the availability of organic substrates through desorption from soil surfaces (Seneviratne & Wild, 1985; Cabrera, 1993) as well as through an increase in the extent of organic surfaces exposed (Birch, 1958; Cabrera, 1993). This suggests that wetting-and-drying cycles are one of the mechanisms by which the soil nitrogen pool is replenished from successively more recalcitrant or physically protected nitrogen pools (Elliot, 1986). It is also argued that alternate wetting and drying can maintain or even increase grain yield because of the enhancement in root growth, grain-filling rate and remobilization of carbon reserves from vegetative tissues to grains All this is consistent with the hypothesis that SRI water management practice of drying and wetting cycles is beneficial to plant growth through an increase in nutrient availability.
Under flooded conditions, despite the fact that ample water is available to the rice plant, there are numerous constraints introduced in terms of nitrogen supply. Lowland rice generally loses more than 60% of applied nitrogen through ammonia volatilization from the floodwater (Ceesay et al., 2006). Microbial activity is reduced, and as a result, the decomposition of soil organic matter is reduced by 50% under anaerobic conditions. Zinc deficiency has been reported as a widespread nutritional disorder in flooded rice. Further, recent research is showing that in continuously flooded rice soils, much of the nitrogen in soil organic matter becomes bonded to aromatic rings and thus is not readily available to the crop (Schmidt-Rohr et al., 2004). The microbial biomass nitrogen is an important repository of plant nutrients that is more labile than the bulk of soil organic matter and able to contribute substantial amounts of nutrients in the soil. Of the factors that contribute to high nitrogen availability and high nitrogen useefficiency under SRI management practices, repeated wetting and drying process may have the greatest influence. SRI‘s water management practices of intermittent irrigation also help in improving root systems (Bouman et al. 2007). According to Kirk and Soilivas, 1997, 75% of roots of rice plants growing in continuously flooded soil remain shallow, in the top 6cm. CF can also cause degeneration of as much as three-fourths of a rice plant's roots by the flowering stage (Kar et al. 1974). This degenerative physiological process presumably has some limiting effect on rice plant performance (Kirk & Bouldin, 1991). Lack of aeration of the soil affects not only root health and functioning but also the populations of beneficial organisms that contribute to plant nutrition and health. Yanni et al., 2001; Feng et al., 2005; Dazzo and Yanni, 2006 have shown that Diazotrophic rhizobacteria render growth promoting services to rice plants by living within them as endophytes. There is also evidence that phosphorus solubilization and availability are increased by alternate wetting and drying of soil (Turner & Haygarth, 2001; Turner et al., 2006; Oberson et al., 2006). Mycorrhizal associations may also be contributing to plant nutrition with SRI practices as these symbiotic fungi, which require aerobic soil conditions, can greatly increase the volume of soil from which plant roots can acquire nutrients (Sieverding, 1991; Pinton et al., 2000). In more aerobic soils, mycorrhizal fungi can enhance the yields of rice (Solaiman & Hirata, 1997; Ellis, 1998). In Mwea irrigation scheme, yields and increases in the area under rice cultivation are currently constrained by the amount of irrigation water available to support production. There is high demand for water in the entire scheme, thus not all farmers grow their crop during the main rice growing season (August-December). Farmers who grow their crop out of this season (January-April) experience high losses in yields due to unfavorable weather conditions. For sustainable rice production and agriculture in general, ways of minimizing water usage are needed. SRI offers opportunities to raise production while using less water. By reducing the need for and use of agrochemical inputs, it can also contribute to food security and environmental quality. CONCLUSIONS This study has shown that SRI water management practice is capable of producing considerably higher rice yields as well as save on water usage than conventional water management practice. SRI practices can address some key constraints for rice production in Kenya and in many other countries. It can reduce water requirements for production (while increasing yield) hence increase water productivity. FAO, 2006 indicate that a 1% increase in water productivity in food production makes available extra 24 liters of water per day per capita. Investing in agriculture and in agricultural water management, therefore, is an attractive strategy for freeing water for other purposes. Water scarcity is likely to become a more significant problem around the world. Adopting cultivation practices such as SRI that use less water is the way forward. ACKNOWLEDGEMENT This research is being supported by the JKUAT Innovation Fund, the Mwea Irrigation and Agricultural Development Center, and farmers in the Mwea scheme. I thank them and my supervisors very much for their inputs to this study. Authors acknowledge the immense help received from the scholars whose articles are cited and included in references of this manuscript. The authors are also grateful to authors/editors/publishers of all those articles, journals and books from where the literature for this article has been reviewed and discussed.
References:
1. American Society for Testing and Materials. Standard test method for open channel flow measurement of water with the Parshall flume. ASTM 1991; D: 1941-91.
2. Bera A. A magic wand for hungry stomachs. Tehelka Magazine 2009; 6(18).
3. Berkelaar D. SRI, the System of Rice Intensification: Less can be more. ECHO Development Notes 2001; 10(70):1-7.
4. Birch HF. The effect of soil drying on humus decomposition and nitrogen. Plant and Soil 1958; 10: 9-31.
5. Bouman BA, Tuong TP. Field water management to save water and increase its productivity in irrigated lowland rice. Agricultural Water Management 2000; 1615:1-20.
6. Bouman BA, Peng S, Castañeda AR, Visperas RM. Yield and water use of irrigated tropical aerobic rice systems. Agricultural Water Management 2005; 74:87-105.
7. Cabrera ML. Modeling the flush of N mineralization caused by drying and rewetting soils. Soil Science Society of America Journal 1993; 57: 63-66.
8. Ceesay M, Reid W, Fernandes E, Uphoff N. The effects of repeated soil wetting and drying on lowland rice yield with System of Rice Intensification (SRI) methods. International Journal of Agricultural sustainability 2006; 4 (1): 5-14.
9. Dazzo FB, Yanni YG. The natural rhizobium-cereal crop association as an example of plant bacterial interaction. In. Uphoff N, Ball A, Fernandes ECM, Herren H, Husson O, Laing M, Palm CA, Pretty J, Sanchez PA, Sanginga N, Thies J. eds. Biological Approaches to Sustainable Soil Systems. Boca Raton: FL. CRC Press; 2006. P. 109-127.
10. D?bereiner J. Biological nitrogen fixation associated with sugar cane and rice. Contributions and prospects for improvement. Plant and Soil 1995; 174: 195-209.
11. Elliot ET. Aggregate structure and carbon, nitrogen, and phosphorus in native and cultivated soils. Soil Science Society of America Journal 1986; 50: 627-633.
12. Ellis JF. Post-flood syndrome and vesiculararbuscular mycorrhizal fungi. Journal of Production Agriculture 1998; 11: 200-204.
13. FAO. Raising water productivity. Spotlight Magazine Rome FAO 2006; AG21.
14. Fen C, Shen SH, Cheng HP, Jing YX, Yanni YG Dazzo FB. Ascending migration of endophytic rhizobia, from roots to leaves, inside rice plants and assessment of benefits to rice growth physiology. Applied and Environmental Microbiology 2005; 71: 7271-7278.
15. Gomez KA, Gomez AA. Statistical Procedures for Agricultural Research. 2nd ed. Wiley (NY): published for the International Rice Research Institute; 1984.
16. Herschy N, Reginald W. Stream flow Measurement. E & FN Spon. An imprint of Chapman and Hall. 2ed. 1995.
17. Inubushi, K Wada H. Easily decomposable organic matter in paddy soils, VII: Effect of various pretreatments on N mineralization in submerged soils, Tokyo. Soil Science and Plant Nutrition 1987; 33: 567-576.
18. Kar S, Varade SB, Subramanyam TK, Ghildyal BP. Nature and growth pattern of rice root system under submerged and unsaturated conditions, Italy. Il Riso 1974; 23:173-179.
19. Kirk GJD, Bouldin DR. Speculations on the operation of the rice root system in relation to nutrient uptake. In. Penning de Vries FWT et al. eds. Simulation and Systems Analysis for Rice Production. Wageningen: Pudoc; 1991; 195-203.
20. Kirk GJD, Solivas JL. On the extent to which root properties and transport through the soil limit nitrogen uptake by lowland rice. European Journal of Soil Science 1997; 48: 613-621.
21. Laulanié H. Le système de riziculture intensive malgache. Tropicultura 1993; 11: 110-114.
22. Magdoff FR, Bouldin DR. Nitrogen fixation in submerged soil-sand-energy material media and the aerobicanaerobic interface. Plant and Soil 1970; 33: 49-61.
23. Mati BM, Nyamai M. Promoting the System of Rice Intensification in Kenya: Growing more with less water [Brochure] 2009.
24. Mati BM, Wanjogu RK, Odongo B, Home PG. Introduction of System of Rice Intensification in Kenya: Experiences from Mwea Irrigation Scheme. Paddy and Water Environment 2011; 9 (1): 145-154.
25. McDonald AJ, Hobbs PR Riha SJ. Does the System of Rice Intensification out-perform conventional best management? A synopsis of the empirical record. Field Crops Research 2006; 96:31-36.
26. Mishra A. System of rice intensification (SRI). A quest for interactive science to mitigate the climate change vulnerability. Asian Institute of Technology, Agricultural Systems and Engineering; School of Environment, Bangkok,Thailand. IOP Conf. Series. Earth and Environmental Science 2009; (6) 24: 20-28.
27. Oberson A, Bunemann EK, Friesen DK, Rao IM, Smithson PC, Turner BL, Frossard E. Improvising phosphorus fertility in tropical soils through biological interventions. In. Uphoff N, Ball A, Palm C, Fernandes E, Pretty J, Herren H, Sanchez P, Husson O, Laing M, Sanginga N, Laing M, Thies J. eds. Biological Approaches to Sustainable Soil Systems. Boca Raton: FL. CRC Press; 2006. P. 531-546.
28. Pinton R, Varanini Z, Nannipieri P. eds. The Rhizosphere: Biochemical and Organic Substance at the Soil-Plant Interface. New York: Marcel Dekker; 2000.
29. Prasad SC. Rethinking innovation and development: Insights from the System of Rice Intensification (SRI) in India. The Innovation Journal: The Public Sector Innovation Journal 2007; 12 (2) 3: 25.
30. Prasad S, Ravindra A. South-South Cooperation and the System of Rice Intensification (SRI). SRI presentation to Kenyan friends during the first National workshop on SRI in Kenya, Nairobi. 2009
31. Republic of Kenya. National Rice Development Strategy 2008-2018. Ministry of Agriculture, Nairobi 2008.
32. Sarath P, Thilak B editors. Comparison of productivity of System of Rice Intensification and conventional rice farming systems in the Dry-Zone Region of Sri Lanka. Proceedings of the 4th International Crop Science Congress of New directions for a diverse planet: 2004 Sep 26-Oct 1; Brisbane, Australia. ISBN 1 920842; 2004; 20: 9
33. Schmidt-Rohr K, Mao JD, Olk DC. Nitrogen-bonded aromatics in soil organic matter and their implications for a yield decline in intensive rice cropping. Proceedings of the National Academy of Science 2004; 101: 6351- 6354.
34. Seneviratne R, Wild A. Effect of mild drying on the mineralization of soil nitrogen. Plant and Soil 1985; 84: 175- 179.
35. Sieverding E. Vesicular-Arbuscular Mycorrhiza Management in Tropical Agro ecosystems. Bremen. Deutsche Gesellschaft fu¨ r Zusammenarbeit; 1991.
36. Soejima H, Sugiyama T, Ishihara K. Changes in the chlorophyll contents of leaves and in levels of cytokinins in root exudates during ripening of rice cultivars Nipponbare and Akenohoshi. Plant Cell Physiology 1995; 36: 1105- 1114.
37. Solaiman MZ, Hirata H. Responses of directly seeded wetland rice to arbuscular mycorrhizal fungi inoculation. Journal of Plant Nutrition 1997; 20: 1479-1487.
38. Sombroek WG, Braun HMH, van der Pouw BJA. The exploratory soil map of Kenya and agro climatic zone map of Kenya scale 1:1million. Exploratory Soil Survey Report No.E1. Kenya Soil Survey, Nairobi, 1982.
39. Stoop WA, Uphoff N, Kassam A. A review of agricultural research issues raised by the system of rice intensification (SRI) from Madagascar: opportunities for improving farming systems for resource-poor farmers. Agricultural Systems 2002; 71: 249- 274.
40. Thakur AK, Uphoff N, Antony E. An assessment of physiological effects of System of Rice Intensification (SRI) practices compared with recommended rice cultivation practices in India. Experimental Agriculture 2009; 46 (1): 77-98.
41. Thakur KA. Critiquing SRI criticism: beyond skepticism with empiricism. Current Science 2010; 98:10.
42. Thakur K A, Rath S Patil DU. Effects on rice plant morphology and physiology of water and associated management practices of the system of rice intensification and their implications for crop performance. Paddy and Water Environment 2011; 9 (1): 13-24.
43. Turner BL, Haygarth PM. Phosphorus solubilization in rewetted soils. Nature 2001 May 17; 411: 258.
44. Turner BL, Frossard E, Oberson A. Enhancing phosphorus availability in low-fertility soils. In. Uphoff N, Ball A, Palm C, Fernandes E, Pretty J, Herren H, Sanchez P, Husson O, ,Sanginga N, Laing M, Thies J. eds. Biological Approaches to Sustainable Soil Systems. Boca Raton: FL. CRC Press; 2006. P. 191-205.
45. Uphoff N. Higher yields with fewer external inputs? The system of rice intensification and potential contributions to agricultural sustainability. International Journal of Agricultural Sustainability 2003; 1:38- 50.
46. Uphoff N, Kassam A. Case study: System of Rice Intensification, in agricultural technologies for developing countries. Final report. Annex 3. European Technology Assessment Group, Karlsruhe, Germany 2009.
47. Vermeule M. More From Less, From Less to More. Scaling Up: Dissemination of a rice cultivation technique. Amsterfoort, the Netherlands. Farming Matter 2009. P 3.
48. Wanjogu RK, Mugambi G., Adoli, H.L., Shisanya, S.O. and Tamura M. [Mwea Rice Production Manual]. Mwea Irrigation Agricultural Development Project. National Irrigation Board library, Nairobi. 1995.
49. World Bank Institute. System of Rice Intensification (SRI): Achieving more with less - A new way of rice cultivation. Overview of SRI - Improving Rice Productivity and Achieving Water Savings. WBI Multimedia Team. Washington, D.C. The Institute; 2008
50. Yanni YG, Rizk RY, Abd El-Fattah FK, Squartini A, Corich V, Giacomini A, de Bruijn F, Rademaker J, MayaFlores J, Ostrom P, Vega-Hernandez M, Hollingsworth RI, Martinez-Molina E, Mateos P, Velazquez E, Wopereis J, Triplett E, Umali-Garcia M, Anarna JA, Rolfe JA, Ladha JK, Hill J, Mujoo R, Ng PK, Dazzo FB. The beneficial plant growth-promoting association of Rhizobium leguminosarum bv. trifolii with rice roots. Australian Journal of Plant Physiology 2001; 28: 845-870.
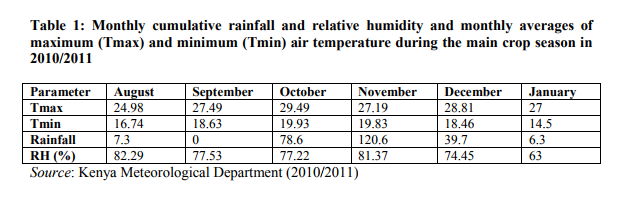
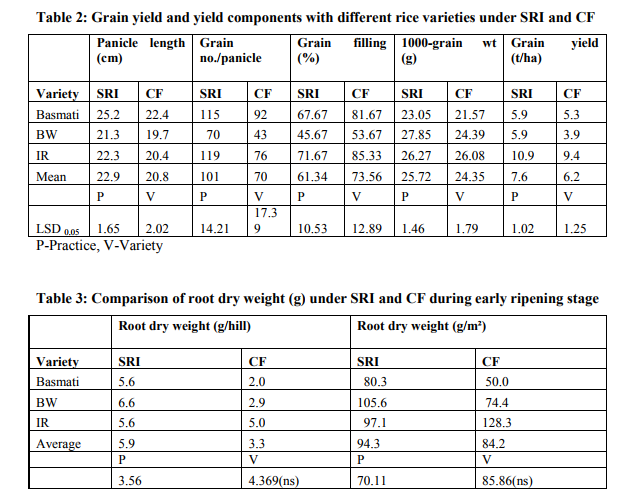
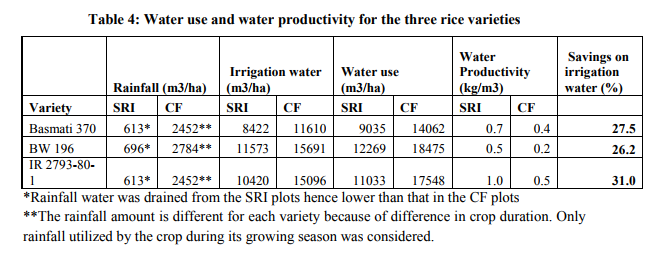
|