IJCRR - 8(21), November, 2016
Pages: 38-46
Print Article
Download XML Download PDF
INVESTIGATION ON THE SOLAR CYCLE SIGNATURE ON THE HADLEY CIRCULATION BASED ON THE INTENSITY AND DURATION OF THE SOLAR CYCLE
Author: C. Vedavathi, M. Venkat Ratnam, V.V.M. Jagannadha Rao, N. Venkwateswara Rao, and S. VijayaBhaskara Rao
Category: General Sciences
Abstract:It is well known that there are profound effects of solar cycle (SC) on the tropical deep convection and hence the atmospheric circulations. However, it is unknown how the intensity and duration of SC is going to affect the circulation patterns. In the present study, the effect of SC on the Hadley circulation (HC) is investigated based on intensity and duration of the SC using ERA-Interim dataset obtained during 1979-2012. Maximum and minimum SC is differentiated based on sunspot number (SSN) with cut-off at >=100 and
Keywords: Solar cycle, Convection, Hadley circulation
Full Text:
Introduction
Tropical latitudes are the prime regions for atmospheric general circulations due to the existence of active convective centres[1]. Among these, prominent are Hadley (meridional or N-S) and Walker (zonal or E-W) circulations. Interestingly, both these circulations have common source region in the tropical latitudes. While the Hadley Circulation (HC) is mainly due to pressure gradient between the land and ocean, the Walker Circulation (WC) is due to the temperature difference between the east and western Pacific oceans[2,3]. The characteristics of these circulations vary (upward and downward branches) from season to season. The HC is dominated by a strong winter hemisphere cell and a very weak summer hemisphere cell [4]. The direction of the HC will be reversed from summer to winter season, and is dominant in the winter hemisphere[4,5]. From December to March, the HC extends roughly between 10oS and 30oN dominant by intense Northern Hemisphere (NH) Hadley cell, whereas during June to September it extends between 10oN and 35oS in Southern Hemisphere (SH) Hadley cell[6].Over the Indian region, the direction of HC during monsoon season is opposite to that in winter[6,7].
Apart from seasons, other phenomena like El Niño–Southern Oscillation (ENSO) and solar cycle (SC) strongly modulate the strength and direction of the circulations[8,9]. Thus, strong inter-annual variability is expected in these circulations. It is well known that SC affects these circulations both directly and indirectly [10,11]. Several studies are carried out using different data sets to examine the role of SC on these circulations. When sun is more active, stronger Hadley cell was observed [12],using vertical velocities from NCEP re-analysis dataset [12]. Similar dependence of the Hadley cell strength on the solar activity was observed [13,14]. During solar maximum condition, suppression of near equatorial convective activity and enhanced off equatorial convection in the Indian monsoon region was noted [15]. Using NCEP zonal mean temperature and zonal wind data a weakened and broadened Hadley cell under solar maximum conditionswas noted [16,17].Again using vertical velocities, poleward expansion of the HC during solar maximum with stronger ascending motions at the edge of the rising branch was observed [18]. [14]and[19]found a strengthened WC during higher solar activityand[20] also found strengthening of the WC during solar maximum in SC 21 and SC 22. The associated sea surface temperature response during solar maximum is to cool anomaly in the equatorial eastern pacific and pole ward shift of Inter Tropical Convergence Zone (ITCZ) and South Pacific Convergence Zone (SPCZ)[14,19]. A clear modulation of the ENSO which is manifested mainly in the western extent of the Walker cell and links to the behaviour of the Indian monsoon is also clearly found [9].
Simplified global circulation models(GCM) provide some indications of the variation in HC and WC by the solar activity on how these responses arise[2,8,21,22].All model runs in which thermal perturbations were applied only in the lower stratosphere show effects throughout the troposphere, with the vertically banded anomalies in the temperature and zonal wind, and changes in the tropospheric mean circulation[10], which is typical of the results of data analysis. Heating the lower stratosphere increases the static stability in this region, lowers the tropopause, and reduces the wave fluxes[17]. Experiment with simplified GCM suggests that the transfer of solar signal to lower levels from stratosphere is due to secondary temperature maximum in the equatorial lower stratosphere [10].
Several previous studies observed the strengthening of tropical circulations during solar maximum [12,13,14,18]while some studies indicate that HC was weakened and broadened to the pole ward region[15,16,17,23]. In general, during high solar activity, larger convective activity is expected which results in to active circulation[24]. Whether this is true for all the solar cycles is not known. Further, how intensity and duration of solar cycle affects the circulation is not understood to the best of our knowledge. Thus, in the present communication, SC effects on the HC are investigated by considering the duration and intensity of the SC using the data covering three SCs (SC21 to SC 23).
Database and methodology
ERA-Interim data products
ERA-Interim is a re-analysis of the global atmospheric data archived at ECMWF (European Center for Medium Range Weather Forecasts). This data is available since 1979 at 0.125o x 0.125o latitude-longitude grids with more (37) pressure levels than the ERA-40 and 15-yr ECMWF Re-analysis (ERA-15) data sets. More details of model development can be obtained from[25]. For the present study, the meridional and vertical wind data from 1979 to 2012 available at 6 h intervals for 37 pressure levels between 1000 hPa and 1 hPa is used.
Sunspot number (SSN)
To represent the solar activity, several types of indicators are available which includes sunspot number (SSN), solar diameter, solar radio flux at 10.7 cm and geomagnetic activity index. The SSNis one of the best known indicators of the solar activity, which has been recorded since the early 17th century. In the present study, we use the monthly mean SSN during 1979-2012 obtained from http://www.ngdc.noaa.gov.
Outgoing Long-wave Radiation (OLR)
We also make use of OLR, which is considered as proxy for tropical deep convection. Monthly mean gridded OLR available during 1979 to 2012 at 2.5o x 2.5o latitude-longitude grids from NOAA climate diagnostics center web site (http://www.cdc.noaa.gov) is used in the present study. Since the main aim is to investigate the SC influence, we made use of the monthly mean OLR data.
El Niño–Southern Oscillation (ENSO) Index
The Oceanic Niño Index (ONI) is one measure of the ENSO and other indices can confirm whether features consistent with a coupled ocean-atmosphere phenomenon accompanied these periods[26].Periods of ENSO Index was based on a threshold of +/- 0.5oC for the ONI (3 month running mean of ERSST.v4 SST anomalies in the Nino 3.4 region (5oN-5oS, 120-170oW)), based on centred 30-year base periods updated every 5 years. ENSO index used in the present study is downloaded from the web site http://www.cpc.ncep.noaa.gov/products/analysis_monitoring/ensostuff/ensoyears.shtml.
Methodology
To estimate the HC,we calculate the mass stream function (MSF). In general MSF is commonly known as meridional overturning circulation (further known as Hadley, Ferrel, Polar cells depending on the latitudes) with unit 109 Kg/s. The meridional over turning velocities are related to MSF (ψ) by υ(meridional wind) and w(vertical wind). MSF ψis computed using pressure coordinates from the ERA-Interim dataset. It is determined by normalization of the inverse gravity and latitudinal belt using the equation

By defining MSF (ψ) as a vertically integrated northward mass flux at latitude θ from pressure level p to the top of the atmosphere, the analytical solution for v can be obtained as
.
Results
Solar cycle intensity and duration
As the present study mainly depends on the intensity of the SC, the classification of the maximum and minimum periods of SC is discussed first. The monthly mean SSN observed during the period of 1976–2012 along with annual mean is shown in Figure 1. There are three SCs (SC 21, SC 22 and SC 23) in the periodconsidered here. Though period and amplitude of SC varies from one cycle to another, it is well known that the average period is 11years. After careful analysis in our earlier publication[27], we considered time period during which the magnitude of SSN exceeding 100 as solar maximum periods and less than 20 as solar minimum periods as shown with green lines in Figure1. Note that thisis an arbitrary choice of a threshold to identify solar maximum and minimum, and main results will not be affected by changing the threshold of 80 (maximum) and 40 (minimum) used by [28], except change in the duration of maximum and minimum periods.This classification is quite different from that of[28], where they removed the climatological mean from the solar maximum which was defined with SSN>80. In comparison, the cut-offs used in this study are more robust to identify the solar maximum and minimum periods as already discussed in[27]. With these cut-offs, the time periods that fall under solar maximum (minimum) for the SC 21, SC 22 and SC 23 are September 1978 to October 1982 (June 1985 to October 1986), August 1988 to June1992 (April 1995 to March 1997), and August 1999 to April 2002 (April2006toJune2010), respectively.
Table 1 shows the duration, intensity and number of months that fall in solar maximum and minimum periods. From the table it is clear that there are 10.9 years, 9.7 years and 12.6 years in SC 21, SC 22 and SC 23, respectively. Interestingly, SC 23 duration is more than average SC period and this is the longest (extended minimum) SC that occurred in this century. The maximum (peak) SSN is observed to be 164.1, 158 and 120.8 in SC 21, SC 22 and SC 23, respectively, which shows a gradual decrease. The number of months that fall under solar maximum (minimum) periods are 50 (17), 47 (24) and 33 (51) months, respectively. Thus, it is clear that duration of solar maximum (minimum) is decreasing (increasing) from SC 21 to SC 23 and the intensity decreases from SC 21 to SC 23. It will be interesting to see the changes in the HC with respect to the SC intensity and duration. Since the HC depends on the deep convection, first we show how tropical convection is organised during different SC conditions.
Solar cycle effect on the tropical convection
To investigate the SC dependency on the tropical deep convection, we considered OLR as a proxy for convection. Figure 2a shows the global distribution of climatological mean OLR observed during 1979 to 2012. Low OLR indicates deep convection. In general, deep convection centres prevail along the equator over African, Indonesian and South American regions near equator. In order to understand the role of SC on the convective activity, difference in the OLR between solar maximum and minimum periods of SC 21 to SC 23 is shown in Figure 2b. Negative (positive) OLR indicates deep (shallow/no) convection. During solar maximum, convection shifts southward over South American and African (10oS) sectors and no convection over Indian and adjoining sectors. Interestingly, heating centre at equator shifted towards west pacific (150o to 180o longitude). If we clearly observe the Indonesian deep convection in figure 2a, it is puzzling to see that it is divided into two parts in difference of maximum and minimum periods of solar cycles. one shifted towards southward to Australia and another towards east i.e., to western Pacific regions. That means these two heating centres might be shifted to above locations by two different circulations, one due to HC (southward) and another due to WC (eastward –west pacific). It is possible that the ITCZ and SPCZ reinforce and lead for strong heating over those areas. The associated SST response during solar maximum is the existence of cool anomaly in the equatorial eastern pacific and pole ward shift of ITCZ and SPCZ[14,19].
Interestingly, the shift in the convective activity is not same and varies significantly from cycle to cycle which is shown in Figure 3. Figure 3 shows the difference in OLR between solar maximum and minimum in each of SC 21, SC 22 and SC 23. Again, negative (positive) OLR indicates deep (no) convection. In SC 21 and SC 22, no convection is observed over Indian and Indonesian sectors and deep convection exists over the pacific region, whereas, in SC 23(extended minimum) quite contrasting features are noticed i.e., deep convection over southern Indian Ocean, Indonesian region and less convection over the pacific region. Precipitation data set also supports the contrasting feature observed (figure not shown) in SC 23. During SC 21, high convection appeared over Pacific region, Australian continent, South America and South Africa sectors and less convection appeared from equator to north Indian Ocean and North West pacific region. This shift in the convection pattern will strongly influence the HC, as the upward branch of HC will be located over deep convection centres.
During SC 21, southward shift deep convection towards northward and eastward shift of Walker means towards western pacific is observed similar to the climatological picture shown in Figure 2a. In SC 22, only shift in WC towards western Pacific is seen and no southward shift of HC is observed. During SC 23, there is only southward shift of HC and no shift in the WC. An additional feature noted is that besides this shift to Australian region, another deep convection persists over the Indonesian region. Note that ENSO will strongly influence these convection patterns and hence HC and WC.
If we correlate the SC intensity and duration with the convection, it is clear that during SC 21, when the intensity (164.1) is more with longer maximum SSN months (50) with average duration of 10.9 years, both zonal and meridional shift in convection is noticed. Whereas during SC 22, intensity (158) is more with longer maximum SSN months (47) with average duration of 9.7 years, only zonal shift in convection centres is noticed. During SC 23, intensity (120.8) is small with smaller maximum SSN and with average duration of 12.6 years, only meridional shift is seen. Thus, it is clear that SC with larger intensity cause the convection centres shift to Pacific i.e. zonally and longer duration in SC cause shift to SH i.e. meridionally. Earlier it is reported that the longer duration SC with lesser number of SSN causes low heating of earth[29].
In order to quantify the SC effects on the HC, we estimated the MSF for each cycle separately after removing the ENSO contribution i.e., without ENSO affected months. MSF estimated during SC 21, SC 22 and SC 23 maximum and minimum conditions is shown in Figure 4. The contours are (± 0.05, 0.1, 0.15, 0.2, 0.4, 0.8, 1.0) × 109kg s−1 with red (blue) colour indicating positive (negative) values. Following the definition of MSF, the red (or positive contours) denote clockwise flow, while the blue (or negative contours) denote counter clock-wise flow. During SC 21 minimum, there are two Hadley cells one in NH and another in SH with rising motion slightly shifting to north of 5oin NH which peaks at 500 hPa. NH HC is strong compared to the SH one but its width is less compared to SH counterpart.
During SC 21 maximum, the NH HC is intensified and its width increased significantly. The upward branch is shifted to slightly to SH around 2oS. The SH HC is shifted to further south but weakened both in intensity and width. This SH HC shift with rising branch seems to coincide with deep convection centre shown in Figure 3 over South Africa (15oS), Australia (15oS) and South America (15oS).
During SC 22 maximum, the HC is strengthened and not much shift in the circulation is observed. This exactly coincides with the OLR difference shown in Figure 3. The deep convection shifted towards western Pacific and no southward shift is observed.The increased rising motion near equator might be due to coincidence of Walker and Hadley upward branches (or collocation of rising branches).
During SC 23,a similar feature of SC 21 is observed. If we compare the circulations during solar maximum of three cycles, it is clear that the NH HC is relatively weak during SC 21 and SC 23 compared to SC 22. There is no SH HC in SC 22. This is interesting when compared the same with the circulations during solar maximum, i.e., the NH circulations during SC 21 and SC 23 intensified in strength and width and also shift to SH as compared to the SC 22 and also vertical extension of HC upto 200 hPa is noticed.
To understand the exact difference in the HC between solar maximum and minimum conditions, difference in the MSF observed during SC 21, SC 22 and SC 23 is shown in Figure 5. The contours are (± 0.05, 0.1, 0.15, 0.2, 0.4, 0.8, 1.0) × 109kg s−1 with red (blue) colour indicating positive (negative) values. From the difference in the MSF, it is clear that during the SC 21 and SC 23, there is an enhancement in the upper level HC whereas no such feature exists in SC 22. Careful observation prompts us to follow that the strong SH HC present during solar minimum in SC 21 and SC 23 is absent during solar maximum whose rising branch contributes to upper level HC. Since the SH HC during SC 22 is weak there by no contribution to HC.
Comparing the shift in the HC and the deep convection centres,we can conclude that the southward shift of NH HC and weakening of SH HC interact in such a way to strengthen the NH Hadley circulation at upper level and shifting to SH i.e., meridional shift. During SC 22, there is only zonal shift in the deep convection to the western pacific andwe see corresponding absence of enhancement in MSF. It is to be noted that the solar influence on the HC is only prominent at upper levels which can be seen from MSF difference.
As the MSF is estimated over zonal means, there may be chance that the signals get cancelled or enhanced due to large spatial variation. In order to bring out the spatial variability, we select a few regions (Indian, Indonesian and Pacific) that are shown with open circles in Figure 3. All the above mentioned analysis is repeated for each of these sectors. MSF observed during SC 21, SC 22 and SC 23 maximum and minimum conditions over Pacific region (174-186oE) is shown in Figure6. Note that ENSO months are not considered in this analysis. Observing each panel indicates that the NH HC is intensified during SC 21 and SC 22 maximum. In SC 23 minimum, both SH and NH HC intensified but NH HC width has increased.
Indonesia region is the common point to HC and WC.Note that these circulations will be quite different during NH winter and summer months. In order to see the SC influence more clearly on the HC, meridional and vertical wind profiles observed over Indonesian region (0oN,120oE) during solar maximum and minimum conditions in the month of July are shown in Figure 7. Note that ENSO affected months are not considered in this analysis. In order to find the effect of ENSO on these circulations, the difference in the profiles of meridional and vertical wind between ENSO and without ENSO months (July) is also superimposed in the same panels of Figure 7. In only SC 23 maximum and SC 22 minimum no ENSO affected July months are there.
During July month, winds become weak northward in the lower level and southward in the upper level over Indonesia. In this region, always upward wind is observed with northward wind and southward wind in the lower and higher altitudes, respectively. This is mainly due to ITCZ shift towards NH and part of SPCZ[14]. Meridional winds are northward in the lower level and southward in the upper levels. In SC 21, peak southward wind was observed at 200 hPaand differences between with and without ENSO affected profiles show very less magnitudes. Vertical windsare high during SC 23 minimum compared to the SC 21 and SC 22 maximum and minimum conditions. The difference with and without ENSO affected profiles indicate the high magnitudesin SC 21 and SC 22.
Summary and Discussion
In the present study, role of solar cycle (SC) on the Hadley circulation (HC) is explained based on intensity and duration of the solar activity using ERA-Interim reanalysis data sets obtained from 1979 to 2012. In the last three SCs (21, 22 and 23), the duration of solar maximum (minimum) months is found decreasing (increasing) while the intensity decreases from SC 21 to SC 23. Role of SC on the tropical deep convection is investigated first using the NOAA interpolated outgoing longwave radiation (OLR) as a proxy during above mentioned period. During solar maximum, convection shifts southward over South American and African (10oS) sectors and no convection over Indian and adjoining sectors. Over Indonesian region, it is puzzling to see that convection centre is divided into two parts one shifted towards southward to Australia and another towards right i.e., to western Pacific regions. This shift is attributed to the shifting of two heating centres by two circulations namely HC (downward) and Walker circulation (WC) (right side –west pacific). However, this variability is found to differ from cycle to cycle.
Similar variability is also seen in the HC which is represented by estimating the mass stream function (MSF) using ERA-Interim meridional and vertical winds. One striking difference noticed between the SC 21 and SC 22 is decrease in HC during solar maximum in SC 22 against SC 21. Theoretical studies and observations suggest a reduction in HC during solar maximum near equatorial regions[15,16,17]. The above mentioned features during SC 22 support this whereas SC 21 differs. This might be due to occurrence of solar maximum and minimum in two different seasons. During SC 21, peak solar activity occurred around Dec. 1979 whereas solar maximum occurred around Jul. 1989 in SC 22. Moreover during SC 22, there is a consistent peak solar activity for the longer period. Effect of ENSO is found to be strong on the HC similar to that reported earlier where reduced HC during ElNino year 1998 is being observed[30, 31].
The reduction of HC during solar maximum is expected. But this is seen only during SC 22. Overall observations show that during SC 22, the HC decreased compared to SC 21 and SC 23 against increase or no change in HC around pacific region. This might be due to persistence in the high sunspot activity for a larger period with large sunspot number. Moreover the peak activity happened during summer months for SC 22. Northward wind (southerly) during SC 23 increased during solar maximum and minimum compared to SC 21 around pacific region i.e., HC is relatively strong compared to SC 21 which means the effect of solar influence is relatively more in SC 21 compared to SC 23. These can be explained based on intensity and duration of SC. Peak SSN during SC 23 is 120.8 (during Mar. 2000) with duration of 12.6 years. Peak SSN during SC 21 is 164.1 (during Dec. 1979) with duration of 10.9 years. Thus, even though the duration of SC 23 is more (12.6 years), the SSN is less (120.8) compared to SC 21 (10.9 years with peak SSN 164). This strong and less period of solar activity might be the reason for relatively strong influence of solar activity on SC 21 compared to SC 23. In addition, the spotless days are very less during SC 21 (273 days) compared to SC 23 (821 days). The above hypothesis is further strengthened if we see circulation during SC 22. During SC 22 (9.7 years duration with SSN 158) the HC is decreased i.e. influence of solar activity is predominant. Further the spotless days are less (309 days).
SC 23 is long with less intensity and more number of spotless days cause less heating over equatorial regions. Observations of convection centres show that heating is more over land regions in SH compared to the oceanic regions. This indicates that during solar maximum the convection is relatively more over SH land regions. Observations of HC show the broadening of cell and extension towards SH and SH HC present in solar minimum is totally weakened.SC 22 is short and intensified causing strong heating over equatorial regions especially over western pacific. HC which is present in solar minimum in NH is little bit strengthened with no change in the SH Hadley cell exactly coinciding with deep convection over equator. SC 21 which has on average duration of 10.9 years with high intensity (164.1) is a mixed phenomenon of SC22 and SC23 with weak NH HC shifted to southward with weakened SH HC.It is evident that the longer or average duration of SC 21 (10.9 years) and SC23 (12.6 years) show weakening of SH HC whereas short duration of SC22 (9.7 year) doesn’t exhibit such feature indicating that the duration of SC influence circulation and convection.
Conclusion
From the above discussion, it is concluded that, HC is strongly influenced (reduced) during solar maximum which depends further on duration and intensity of SC. Less duration causes more influence on the HC.
Acknowledgements
This work is carried out as a part of CAWSES India Phase-II fully sponsored by ISRO. One of authors (CV) wishes to thank UGC-SVU centre for MST radar application for support. Authors
acknowledge the immense help received from the scholars whose articlesare cited and included in references of this manuscript.The authors arealso grateful to authors / editors / publishers of all those articles, journalsand books from where the literature for this article has been reviewed anddiscussed.
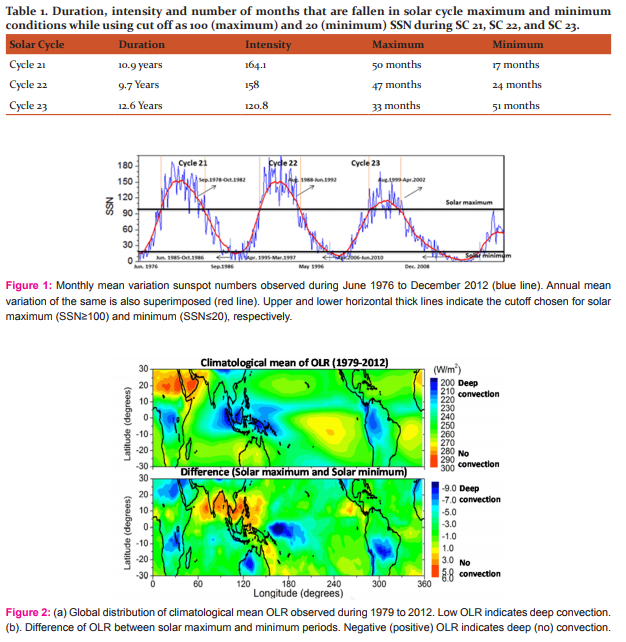
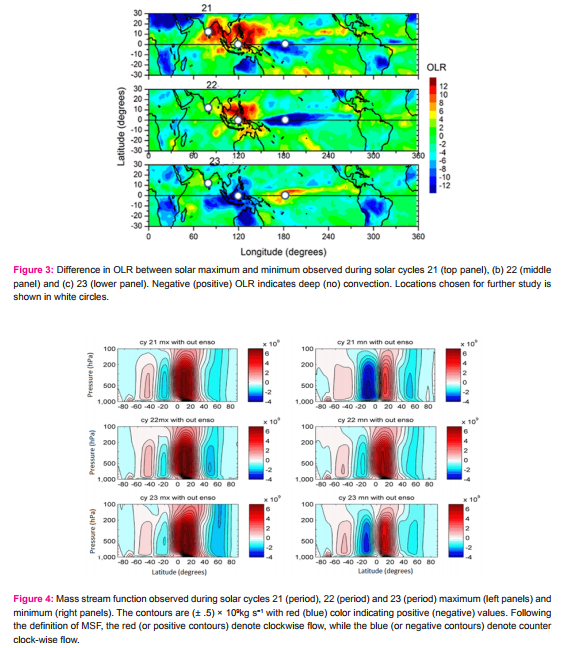
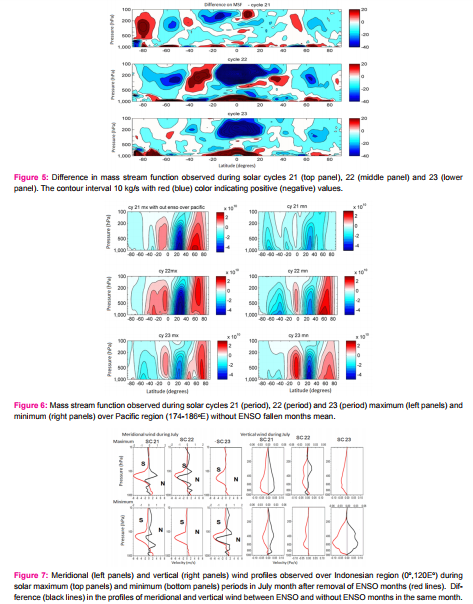
References:
- Annes, V.H, Mohan Kumar, K., Joseph, P.V., 2001. Winter and summer Hadley circulations over Peninsular India as monitored by MST radar at Gadanki (13.47oN, 79.18oE). Inter. J.Climat. 21, 593-601.
- Lau, K-M., Yang, S., 2002. Walker circulation. Elsevier Sciences, doi:10.1006/rwas.2002.0450.
- Tapio Schneider., 2006. General circulation of the Atmosphere, Annu. Rev. Earth Planet. Sci. 2006. 34:655–88.
- Cook. K., 2004. Hadley circulation Dynamics: Seasonality and the Role of Continents. Adv. Global Clim. Cha. Res.,
- Roja Raman. M.,Jagannadha Rao, V.V.M., VenkatRatnam, M., Kishore Kumar, G., Narendra Babu, A., VijayaBhaskara Rao, S., Prabhakara Rao, N., Narayana Rao, D., 2008. Atmospheric circulation during active and break phases of Indian summer monsoon: A study using MST radar at Gadanki (13.5 N, 79.2E). J. Geophys. Res, 113, D20124, doi:10,1029/2008jD010341.
- Oort, A. H., Rasmusson, E.M., 1970. On the annual variation of the monthly mean meridional circulation. Mon. Weather Rev., 98, 423–442, doi:10.1175/1520-0493(1970)098<0423:OTAVOT>2.3.CO;2
- Sikka, D.R., 1980. Some aspects of the largescale fluctuations of summer monsoon rainfall over India in relation to fluctuations in the planetary and regional scale circulation parameters. Proc. Indian Acad. Sci. Earth Planet. Sci. 89:179–95.
- Foley, A., Jonathan, Aure ´lie Botta, and Michael T. Coe., 2002. El Nin ˜o–Southern oscillation and the climate, ecosystems and rivers of Amazonia. Global Biogeo. Cycles, 16, 4, 1132, doi:10.1029/2002GB001872.
- Kodera, K., Coughlin, K., Arakawa, O., 2007. Possible modulation of the connection between the Pacific and Indian Ocean variability by the solar cycle. Geophys. Res. Lett., 34, L03710, doi:10.1029/2006GL027827.
- Gray, L.J., 2010. Solar influences on climate. Rev. Geophys. 48, RG4001, http://dx.doi.org/10.1029/2009RG000282.
- Dubey, S.C., 2005. Solar variability and climate change.Indian J. of Geo-Marine Sci., 43(5), 871-875.
- Labitzke, K., Van Loon, H., 1995. Connections between the troposphere and stratosphere on a decadal scale. Tellus, Ser. A, 47, 275–286, doi:10.1034/j.1600-0870.1995.t01-1-00008.x.
- Van Loon, H., Meehl, G.A., Arblaster, J.M., 2004. A decadal solar effect in the tropics in July–August. J. Atmos. Sol. Terr. Phys., 66, 1767–1778, doi:10.1016/j.jastp.2004.06.003.
- Van Loon, H., Meehl, G.A., Shea, D., 2007. The effect of the decadal solar oscillation in the Pacific troposphere in northern winter. J. Geophys. Res., 112, D02108, doi:10.1029/ 2006JD007378.
- Kodera, K., 2004. Solar influence on the Indian Ocean Monsoon through dynamical processes. Geophy. Res. Lett., 31, L24209, doi:10.1029/2004GL020928.
- Haigh, J. D., 2003. The effects of solar variability on the Earth’s climate, Philos. Trans. R. Soc. London, Ser. A, 361,95–111, doi:10.1098/rsta.2002.1111
- Haigh, J. D., Blackburn, M., Day, R., 2005. The response of tropospheric circulation to perturbations in lower?stratospheric temperature. J. Clim., 18, 3672–3685, doi:10.1175/JCLI3472.1.
- Gleisner, H., Thejll, P., 2003. Patterns of tropospheric response to solar variability. Geophys. Res. Lett., 30(13), 1711, doi:10.1029/2003GL017129.
- Meehl, G. A., Arblaster, J.M., Branstator, G., Van Loon, H., 2008. A coupled air?sea response mechanism to solar forcing in the Pacific region. J. Clim., 21, 2883–2897, doi:10.1175/ 2007JCLI1776.1.
- Lee, J. N., Shindell, D.T., Hameed, S., 2009. The influence of solar forcing on tropical circulation. J. Clim., 22, 5870–5885, doi:10.1175/2009JCLI2670.1.
- Chunzai Wang., 2002. ENSO and Atmospheric circulation cells. CLIVAR Exchanges. 7, 2, 9-11.
- Gastineau, Guillaume., Li, Laurent., Le Treut, Hervé., 2009. The Hadley and Walker circulation changes in global warming conditions described by idealized atmospheric simulations. J. Clim., 22, 14, 3993-4013.
- Bronnimann, S., Ewen, T., Griesser, T., Jenne, R., 2006. Multidecadal signal of solar variability in the upper troposphere during the 20th century. Space Sci. Rev., 125, 305-317.
- Fleagle, Rabert, G., Joost, A. Businger., 1980. An Introduction to atmospheric physics. Second edition, Acadamic Press.
- Simmons, A.J., Willett, K.M., Jones, P.D., Thorne, P.W., Dee, D.P., 2010. Low-frequency variations in surface atmospheric humidity, temperature, and precipitation inferences from re-analyses and monthly grided observational data. J. Geophys.Res. 115, D01110, http://dx.doi.org/10.1029/2009JD012442.
- Trenberth, Kevin E., 1997. The Definition of El Niño. Bull. Amer. Meteor. Soc. 78, 12, 2771-2777., 10.1175/1520-0477(1997)078<2771:TDOENO>2.0.CO;2
- VenkatRatnam M., Venkateswara Rao. N., Vedavathi, C., Krishna Murthy. B.V., VijayaBhaskara Rao, S., 2014. Diurnal tide in the low-latitude troposphere and stratosphere: Long-term trends and role of the extended solar minimum. J. Atmos. Sol. Terr. Phy., http://dx.doi.org/10.1016/j.jastp.2014.06.0.04.
- Roy.I, Haigh, J., 2012. Solar Cycle Signals in the Pacific and the Issue of Timings, J. Atmos. Sci., 69, 1446-1451, doi: 10.1175/JAS-D-11-0277.1.
- Bhattacharya., 2011. Longer duration solar cycle with lesser number of SSN causes low heating of earth. Inter. J. Eng. Sci. and Tech. 3, 8012-8017.
- Jagannadha Rao, V. V. M., Narendra Babu, A., VijayaBhaskara Rao, S., Narayana Rao, D., 2007. Anomalous wind circulation observed during 1997/98 ElNino using Indian MST Radar. J. Appl. Meteorol. Clima., 46, 112-119.
- Jagannadha Rao, V.V.M., Narayana Rao, D., VenkatRatnam, M., Mohan, K., VijayaBhaskar Rao, S., 2003. Mean Vertical Velocities Measured by Indian MST Radar and Comparison with Indirectly Computed Values. J. App. Meteo., 42 (4), 541-552.
|